From Wikipedia, the free encyclopedia
![]()
Computer-generated map of Pluto[1]
|
|||||||||
Discovery | |||||||||
---|---|---|---|---|---|---|---|---|---|
Discovered by | Clyde W. Tombaugh | ||||||||
Discovery date | February 18, 1930 | ||||||||
Designations | |||||||||
MPC designation | 134340 Pluto | ||||||||
Pronunciation | ![]() |
||||||||
Named after
|
Pluto | ||||||||
Adjectives | Plutonian | ||||||||
Orbital characteristics[6][b] | |||||||||
Epoch J2000 | |||||||||
Aphelion |
|
||||||||
Perihelion |
|
||||||||
|
|||||||||
Eccentricity | 0.244671664 (J2000) 0.248 807 66 (mean)[3] |
||||||||
366.73 days[3] | |||||||||
Average orbital speed
|
4.7 km/s[3] | ||||||||
14.86012204°[5] | |||||||||
Inclination |
|
||||||||
110.28683° | |||||||||
113.76349° | |||||||||
Known satellites | 5 | ||||||||
Physical characteristics | |||||||||
Mean radius
|
|||||||||
|
|||||||||
Volume |
|
||||||||
Mass | |||||||||
Mean density
|
2.03±0.06 g/cm3[9] | ||||||||
1.229 km/s[f] | |||||||||
Sidereal rotation period
|
|
||||||||
Equatorial rotation velocity
|
47.18 km/h | ||||||||
119.591°±0.014° (to orbit)[9][g] | |||||||||
North pole right ascension
|
312.993°[10] | ||||||||
North pole declination
|
6.163°[10] | ||||||||
Albedo | 0.49 to 0.66 (geometric, varies by 35%)[3][11] | ||||||||
|
|||||||||
13.65[3] to 16.3[12] (mean is 15.1)[3] |
|||||||||
−0.7[13] | |||||||||
0.065″ to 0.115″[3][h] | |||||||||
Atmosphere | |||||||||
Surface pressure
|
0.30 Pa (summer maximum) | ||||||||
Composition | Nitrogen, methane, carbon monoxide[14] |
Pluto (134340 Pluto) is the largest object in the Kuiper belt,[i][j] the tenth-most-massive known body directly orbiting the Sun, and the second-most-massive known dwarf planet, after Eris. Like other Kuiper belt objects, Pluto is primarily made of rock and ice,[15] and relatively small, about 1/6 the mass of the Moon and 1/3 its volume. It has an eccentric and highly inclined orbit that takes it from 30 to 49 AU (4.4–7.4 billion km) from the Sun. Hence Pluto periodically comes closer to the Sun than Neptune, but an orbital resonance with Neptune prevents the bodies from colliding. In 2014 it was 32.6 AU from the Sun. Light from the Sun takes about 5.5 hours to reach Pluto at its average distance (39.4 AU).[16]
Discovered in 1930, Pluto was originally considered the ninth planet from the Sun. Its status as a major planet fell into question following further study of it and the outer Solar System over the next 75 years. Starting in 1977 with the discovery of the minor planet Chiron, numerous icy objects similar to Pluto with eccentric orbits were found.[17] The scattered disc object Eris, discovered in 2005, is 27% more massive than Pluto.[18] The understanding that Pluto is only one of several large icy bodies in the outer Solar System prompted the International Astronomical Union (IAU) to formally define "planet" in 2006. This definition excluded Pluto and reclassified it as a member of the new "dwarf planet" category (and specifically as a plutoid).[19] Astronomers who oppose this decision hold that Pluto should have remained classified as a planet, and that other dwarf planets and even moons should be added to the list of planets along with Pluto.[20][21][22]
Pluto has five known moons: Charon (the largest, with a diameter just over half that of Pluto), Nix, Hydra, Kerberos, and Styx.[23] Pluto and Charon are sometimes described as a binary system because the barycenter of their orbits does not lie within either body.[24] The IAU has yet to formalise a definition for binary dwarf planets, and Charon is officially classified as a moon of Pluto.[25]
On July 14, 2015, the Pluto system is due to be visited by spacecraft for the first time.[26] The New Horizons probe will perform a flyby during which it will attempt to take detailed measurements and images of Pluto and its moons.[27] Afterwards, the probe may visit several other objects in the Kuiper belt.[28]
Discovery

In the 1840s, using Newtonian mechanics, Urbain Le Verrier predicted the position of the then-undiscovered planet Neptune after analysing perturbations in the orbit of Uranus.[29] Subsequent observations of Neptune in the late 19th century caused astronomers to speculate that Uranus's orbit was being disturbed by another planet besides Neptune.
In 1906, Percival Lowell—a wealthy Bostonian who had founded the Lowell Observatory in Flagstaff, Arizona, in 1894—started an extensive project in search of a possible ninth planet, which he termed "Planet X".[30] By 1909, Lowell and William H. Pickering had suggested several possible celestial coordinates for such a planet.[31] Lowell and his observatory conducted his search until his death in 1916, but to no avail. Unknown to Lowell, on March 19, 1915, surveys had captured two faint images of Pluto, but they were not recognized for what they were.[31][32] There are fifteen other known prediscoveries, with the oldest made by the Yerkes Observatory on August 20, 1909.[33]
Because of a ten-year legal battle with Constance Lowell, Percival's widow, who attempted to wrest the observatory's million-dollar portion of his legacy for herself, the search for Planet X did not resume until 1929,[34] when its director, Vesto Melvin Slipher, summarily handed the job of locating Planet X to Clyde Tombaugh, a 23-year-old Kansan who had just arrived at the Lowell Observatory after Slipher had been impressed by a sample of his astronomical drawings.[34]
Tombaugh's task was to systematically image the night sky in pairs of photographs, then examine each pair and determine whether any objects had shifted position. Using a machine called a blink comparator, he rapidly shifted back and forth between views of each of the plates to create the illusion of movement of any objects that had changed position or appearance between photographs. On February 18, 1930, after nearly a year of searching, Tombaugh discovered a possible moving object on photographic plates taken on January 23 and January 29 of that year. A lesser-quality photograph taken on January 21 helped confirm the movement.[35] After the observatory obtained further confirmatory photographs, news of the discovery was telegraphed to the Harvard College Observatory on March 13, 1930.[31]
Name
The discovery made headlines across the globe. The Lowell Observatory, which had the right to name the new object, received over 1,000 suggestions from all over the world, ranging from Atlas to Zymal.[36] Tombaugh urged Slipher to suggest a name for the new object quickly before someone else did.[36] Constance Lowell proposed Zeus, then Percival and finally Constance. These suggestions were disregarded.[37]The name Pluto, after the god of the underworld, was proposed by Venetia Burney (1918–2009), an eleven-year-old schoolgirl in Oxford, England, who was interested in classical mythology.[38] She suggested it in a conversation with her grandfather Falconer Madan, a former librarian at the University of Oxford's Bodleian Library, who passed the name to astronomy professor Herbert Hall Turner, who cabled it to colleagues in the United States.[38]
The object was officially named on March 24, 1930.[39][40] Each member of the Lowell Observatory was allowed to vote on a short-list of three: Minerva (which was already the name for an asteroid), Cronus (which had lost reputation through being proposed by the unpopular astronomer Thomas Jefferson Jackson See), and Pluto. Pluto received every vote.[41] The name was announced on May 1, 1930.[38] Upon the announcement, Madan gave Venetia GB£5 (£276 as of 2015),[42] as a reward.[38]
The choice of name was partly inspired by the fact that the first two letters of Pluto are the initials of Percival Lowell, and Pluto's astronomical symbol (



The name was soon embraced by wider culture. In 1930, Walt Disney was apparently inspired by it when he introduced for Mickey Mouse a canine companion named Pluto, although Disney animator Ben Sharpsteen could not confirm why the name was given.[44] In 1941, Glenn T. Seaborg named the newly created element plutonium after Pluto, in keeping with the tradition of naming elements after newly discovered planets, following uranium, which was named after Uranus, and neptunium, which was named after Neptune.[45]
Most languages use the name "Pluto" in various transliterations.[k] In Japanese, Houei Nojiri suggested the translation Meiōsei (冥王星?, "Star of the King(God) of the Underworld"), and this was borrowed into Chinese, Korean, and Vietnamese.[46][47][48] Some Indian languages use the name Pluto, but others, such as Hindi, use the name of Yama, the Guardian of Hell in Hindu and Buddhist mythology, as does Vietnamese.[47] Polynesian languages also tend to use the indigenous god of the underworld, as in Maori Whiro.[47]
Demise of Planet X
Year | Mass | Notes |
---|---|---|
1931 | 1 Earth | Nicholson & Mayall[49][50][51] |
1948 | 0.1 (1/10) Earth | Kuiper[52] |
1976 | 0.01 (1/100) Earth | Cruikshank, Pilcher, & Morrison[53] |
1978 | 0.002 (1/500) Earth | Christy & Harrington[54] |
2006 | 0.00218 (1/459) Earth | Buie et al.[9] |
Astronomers initially calculated its mass based on its presumed effect on Neptune and Uranus. In 1931 Pluto was calculated to be roughly the mass of Earth, with further calculations in 1948 bringing the mass down to roughly that of Mars.[50][52] In 1976, Dale Cruikshank, Carl Pilcher and David Morrison of the University of Hawaii calculated Pluto's albedo for the first time, finding that it matched that for methane ice; this meant Pluto had to be exceptionally luminous for its size and therefore could not be more than 1 percent the mass of Earth.[53] (Pluto's albedo is 1.3–2.0 times greater than that of Earth.[3])
In 1978, the discovery of Pluto's moon Charon allowed the measurement of Pluto's mass for the first time. Its mass, roughly 0.2% that of Earth, was far too small to account for the discrepancies in the orbit of Uranus. Subsequent searches for an alternative Planet X, notably by Robert Sutton Harrington,[55] failed. In 1992, Myles Standish used data from Voyager 2's 1989 flyby of Neptune, which had revised the planet's total mass downward by 0.5%, to recalculate its gravitational effect on Uranus. With the new figures added in, the discrepancies, and with them the need for a Planet X, vanished.[56] Today, the majority of scientists agree that Planet X, as Lowell defined it, does not exist.[57] Lowell had made a prediction of Planet X's position in 1915 that was fairly close to Pluto's position at that time; Ernest W. Brown concluded soon after Pluto's discovery that this was a coincidence,[58] a view still held today.[56]
Orbit and rotation
Pluto's orbital period is 248 Earth years. Its orbital characteristics are substantially different from those of the planets, which follow nearly circular orbits around the Sun close to a flat reference plane called the ecliptic. In contrast, Pluto's orbit is highly inclined relative to the ecliptic (over 17°) and highly eccentric (elliptical). This high eccentricity means a small region of Pluto's orbit lies nearer the Sun than Neptune's. The Pluto–Charon barycenter came to perihelion on September 5, 1989,[2][l] and was last closer to the Sun than Neptune between February 7, 1979, and February 11, 1999. Pluto and
Neptune make their closest approach when it is at 27.960 AU.[59]
In the long term, Pluto's orbit is in fact chaotic. Although computer simulations can be used to predict its position for several million years (both forward and backward in time), after intervals longer than the Lyapunov time of 10–20 million years, calculations become speculative: Pluto is sensitive to unmeasurably small details of the Solar System, hard-to-predict factors that will gradually disrupt its orbit.[60][61] Millions of years from now, Pluto may well be at aphelion, at perihelion or anywhere in between, with no way for us to predict which. This does not mean Pluto's orbit itself is unstable, but its position on that orbit is impossible to determine so far ahead. Several resonances and other dynamical effects keep Pluto's orbit stable, safe from planetary collision or scattering.
Relationship with Neptune

Orbit of Pluto—polar view. This "view from above" shows how Pluto's orbit (in red) is less circular than Neptune's (in blue), and how Pluto is sometimes closer to the Sun than Neptune. The darker halves of both orbits show where they pass below the plane of the ecliptic.
Despite Pluto's orbit appearing to cross that of Neptune when viewed from directly above, the two objects' orbits are aligned so that they can never collide or even approach closely. There are several reasons why.
At the simplest level, one can examine the two orbits and see that they do not intersect. When Pluto is closest to the Sun, and hence closest to Neptune's orbit as viewed from above, it is also the farthest above Neptune's path. Pluto's orbit passes about 8 AU above that of Neptune, preventing a collision.[62][63][64] Pluto's ascending and descending nodes, the points at which its orbit crosses the ecliptic, are currently separated from Neptune's by over 21°.[65]
This alone is not enough to protect Pluto; perturbations from the planets (especially Neptune) could alter aspects of Pluto's orbit (such as its orbital precession) over millions of years so that a collision could be possible. Some other mechanism or mechanisms must therefore be at work. The most significant of these is that Pluto lies in the 2:3 mean-motion resonance with Neptune: for every two orbits that Pluto makes around the Sun, Neptune makes three. The two objects then return to their initial positions and the cycle repeats, each cycle lasting about 500 years. This pattern is such that, in each 500-year cycle, the first time Pluto is near perihelion Neptune is over 50° behind Pluto. By Pluto's second perihelion, Neptune will have completed a further one and a half of its own orbits, and so will be a similar distance ahead of Pluto. Pluto and Neptune's minimum separation is over 17 AU. Pluto comes closer to Uranus (11 AU) than it does to Neptune.[64]
The 2:3 resonance between the two bodies is highly stable, and is preserved over millions of years.[66] This prevents their orbits from changing relative to one another; the cycle always repeats in the same way, and so the two bodies can never pass near to each other. Thus, even if Pluto's orbit were not highly inclined the two bodies could never collide.[64]
Other factors
Numerical studies have shown that over periods of millions of years, the general nature of the alignment between Pluto and Neptune's orbits does not change.[62][67] There are several other resonances and interactions that govern the details of their relative motion, and enhance Pluto's stability. These arise principally from two additional mechanisms (besides the 2:3 mean-motion resonance).First, Pluto's argument of perihelion, the angle between the point where it crosses the ecliptic and the point where it is closest to the Sun, librates around 90°.[67] This means that when Pluto is nearest the Sun, it is at its farthest above the plane of the Solar System, preventing encounters with Neptune. This is a direct consequence of the Kozai mechanism,[62] which relates the eccentricity of an orbit to its inclination to a larger perturbing body—in this case Neptune. Relative to Neptune, the amplitude of libration is 38°, and so the angular separation of Pluto's perihelion to the orbit of Neptune is always greater than 52° (90°–38°). The closest such angular separation occurs every 10,000 years.[66]
Second, the longitudes of ascending nodes of the two bodies—the points where they cross the ecliptic—are in near-resonance with the above libration. When the two longitudes are the same—that is, when one could draw a straight line through both nodes and the Sun—Pluto's perihelion lies exactly at 90°, and hence it comes closest to the Sun at its maximally above Neptune's orbit. This is known as the 1:1 superresonance. All the Jovian planets, particularly Jupiter, play a role in the creation of the superresonance.[62]
To understand the nature of the libration, imagine a polar point of view, looking down on the ecliptic from a distant vantage point where the planets orbit counterclockwise. After passing the ascending node, Pluto is interior to Neptune's orbit and moving faster, approaching Neptune from behind. The strong gravitational pull between the two causes angular momentum to be transferred to Pluto, at Neptune's expense. This moves Pluto into a slightly larger orbit, where it travels slightly slower, according to Kepler's third law. As its orbit changes, this has the gradual effect of changing the perihelion and longitude of Pluto's orbit (and, to a lesser degree, of Neptune). After many such repetitions, Pluto is sufficiently slowed, and Neptune sufficiently speeded up, that Neptune begins to catch up with Pluto at the opposite side of its orbit (near the opposing node to where we began). The process is then reversed, and Pluto loses angular momentum to Neptune, until Pluto is sufficiently speeded up that it begins to catch Neptune again at the original node. The whole process takes about 20,000 years to complete.[64][66]
Rotation
Pluto's rotation period, its day, is equal to 6.39 Earth days.[68] Like Uranus, Pluto rotates on its "side" on its orbital plane, with an axial tilt of 120°, and so its seasonal variation is extreme; at its solstices, one-fourth of its surface is in continuous daylight, whereas another fourth is in continuous darkness.[69]Physical characteristics
Pluto's distance from Earth makes in-depth investigation difficult. Many details about Pluto will remain unknown until 2015, when the New Horizons spacecraft is expected to arrive there.[70]
Appearance and surface
Pluto's visual apparent magnitude averages 15.1, brightening to 13.65 at perihelion.[3] To see it, a telescope is required; around 30 cm (12 in) aperture being desirable.[71] It looks star-like and without a visible disk even in large telescopes, because its angular diameter is only 0.11".The earliest maps of Pluto, made in the late 1980s, were brightness maps created from close observations of eclipses by its largest moon, Charon. Observations were made of the change in the total average brightness of the Pluto–Charon system during the eclipses. For example, eclipsing a bright spot on Pluto makes a bigger total brightness change than eclipsing a dark spot. Computer processing of many such observations can be used to create a brightness map. This method can also track changes in brightness over time.[72][73]
Current maps have been produced from images from the Hubble Space Telescope (HST), which offers the highest resolution currently available, and show considerably more detail,[74] resolving variations several hundred kilometres across, including polar regions and large bright spots.[75] The maps are produced by complex computer processing, which find the best-fit projected maps for the few pixels of the Hubble images.[76] The two cameras on the HST used for these maps are no longer in service, so these will likely remain the most detailed maps of Pluto until the 2015 flyby of New Horizons.[76]
These maps, together with Pluto's lightcurve and the periodic variations in its infrared spectra, reveal that Pluto's surface is remarkably varied, with large changes in both brightness and color.[77] Pluto is one of the most contrastive bodies in the Solar System, with as much contrast as Saturn's moon Iapetus.[74] The color varies between charcoal black, dark orange and white:[78] Buie et al. term it "significantly less red than Mars and much more similar to the hues seen on Io with a slightly more orange cast".[75]
Pluto's surface has changed between 1994 and 2002–3: the northern polar region has brightened and the southern hemisphere darkened.[78] Pluto's overall redness has also increased substantially between 2000 and 2002.[78] These rapid changes are probably related to seasonal condensation and sublimation of portions of Pluto's atmosphere, amplified by Pluto's extreme axial tilt and high orbital eccentricity.[78]
Spectroscopic analysis of Pluto's surface reveals it to be composed of more than 98 percent nitrogen ice, with traces of methane and carbon monoxide.[79] The face of Pluto oriented toward Charon contains more methane ice, whereas the opposite face contains more nitrogen and carbon monoxide ice.[80]
Structure
Observations by the Hubble Space Telescope place Pluto's density at between 1.8 and 2.1 g/cm3, suggesting its internal composition consists of roughly 50–70 percent rock and 30–50 percent ice by mass.[82] Because the decay of radioactive elements would eventually heat the ices enough for the rock to separate from them, scientists expect that Pluto's internal structure is differentiated, with the rocky material having settled into a dense core surrounded by a mantle of ice. The diameter of the core is hypothesized to be approximately 1700 km, 70% of Pluto's diameter.[81] It is possible that such heating continues today, creating a subsurface ocean layer of liquid water some 100 to 180 km thick at the core–mantle boundary.[81][83] The DLR Institute of Planetary Research calculated that Pluto's density-to-radius ratio lies in a transition zone, along with Neptune's moon Triton, between icy satellites like the mid-sized moons of Uranus and Saturn, and rocky satellites such as Jupiter's Io.[84]
Mass and size
Pluto's mass is 1.31×1022 kg, less than 0.24 percent that of Earth,[85] and its diameter is 2306±20 km, or roughly 66% that of the Moon.[9] Its surface area (1.665×107 km2) is about 10% smaller than that of South America. Pluto's atmosphere complicates determining its true solid size within a certain margin.[8] Pluto's albedo varies from 0.49–0.66.
The discovery of Pluto's satellite Charon in 1978 enabled a determination of the mass of the Pluto–Charon system by application of Newton's formulation of Kepler's third law. Once Charon's gravitational effect was measured, Pluto's true mass could be determined. Observations of Pluto in occultation with Charon allowed scientists to establish Pluto's diameter more accurately, whereas the invention of adaptive optics allowed them to determine its shape more accurately.[86]
Year | Radius (diameter) | Notes |
---|---|---|
1993 | 1195 (2390) km | Millis, et al.[87] (If no haze)[88] |
1993 | 1180 (2360) km | Millis, et al. (surface & haze)[88] |
1994 | 1164 (2328) km | Young & Binzel[89] |
2006 | 1153 (2306) km | Buie, et al.[9] |
2007 | 1161 (2322) km | Young, Young, & Buie[8] |
2011 | 1180 (2360) km | Zalucha, et al.[90] |
2014 | 1184 (2368) km | Lellouch, et al.[7] |
Pluto is more than twice the diameter and a dozen times the mass of the dwarf planet Ceres, the largest object in the asteroid belt. It is less massive than the dwarf planet Eris, a trans-Neptunian object discovered in 2005. Given the error bars in the different size estimates, it is currently unknown whether Eris or Pluto has the larger diameter.[88] Both Pluto and Eris are estimated to have solid-body diameters of about 2330 km.[88]
Determinations of Pluto's size are complicated by its atmosphere, and possible hydrocarbon haze.[88] In March, 2014, Lellouch, de Bergh et al. published findings regarding methane mixing ratios in Pluto's atmosphere consistent with a Plutonian diameter greater than 2360 km, with a "best guess" of 2368 km, which would make it slightly larger than Eris.[7]
Atmosphere
Pluto's atmosphere consists of a thin envelope of nitrogen, methane, and carbon monoxide gases, which are derived from the ices of these substances on its surface.[91] Its surface pressure ranges from 6.5 to 24 μbar.[92] Pluto's elongated orbit is predicted to have a major effect on its atmosphere: as Pluto moves away from the Sun, its atmosphere should gradually freeze out, and fall to the ground. When Pluto is closer to the Sun, the temperature of Pluto's solid surface increases, causing the ices to sublimate into gas. This creates an anti-greenhouse effect; much as sweat cools the body as it evaporates from the surface of the skin, this sublimation cools the surface of Pluto. In 2006, scientists using the Submillimeter Array discovered that Pluto's temperature is about 43 K (−230 °C), 10 K colder than would otherwise be expected.[93]
The presence of methane, a powerful greenhouse gas, in Pluto's atmosphere creates a temperature inversion, with average temperatures 36 K warmer 10 km above the surface.[94] The lower atmosphere contains a higher concentration of methane than its upper atmosphere.[94]
Evidence of Pluto's atmosphere was first suggested by Noah Brosch and Haim Mendelson of the Wise Observatory in Israel in 1985,[95] and then definitively detected by the Kuiper Airborne Observatory in 1988, from observations of occultations of stars by Pluto.[96] When an object with no atmosphere moves in front of a star, the star abruptly disappears; in the case of Pluto, the star dimmed out gradually.[95] From the rate of dimming, the atmospheric pressure was determined to be 0.15 pascal, roughly 1/700,000 that of Earth.[97]
In 2002, another occultation of a star by Pluto was observed and analysed by teams led by Bruno Sicardy of the Paris Observatory,[98] James L. Elliot of MIT,[99] and Jay Pasachoff of Williams College.[100] Surprisingly, the atmospheric pressure was estimated to be 0.3 pascal, even though Pluto was farther from the Sun than in 1988 and thus should have been colder and had a more rarefied atmosphere. One explanation for the discrepancy is that in 1987 the south pole of Pluto came out of shadow for the first time in 120 years, causing extra nitrogen to sublimate from the polar cap. It will take decades for the excess nitrogen to condense out of the atmosphere as it freezes onto the north pole's now continuously dark ice cap.[101] Spikes in the data from the same study revealed what may be the first evidence of wind in Pluto's atmosphere.[101] Another stellar occultation was observed by the MIT-Williams College team of James L. Elliot, Jay Pasachoff, and a Southwest Research Institute team led by Leslie A. Young on June 12, 2006, from sites in Australia.[102]
In October 2006, Dale Cruikshank of NASA/Ames Research Center (a New Horizons co-investigator) and his colleagues announced the spectroscopic discovery of ethane on Pluto's surface. This ethane is produced from the photolysis or radiolysis (i.e. the chemical conversion driven by sunlight and charged particles) of frozen methane on Pluto's surface and suspended in its atmosphere.[103]
Satellites
Pluto has five known natural satellites: Charon, first identified in 1978 by astronomer James Christy; Nix and Hydra, both discovered in 2005,[104] Kerberos, discovered in 2011,[105] and Styx, discovered in 2012.[106]The Plutonian moons are unusually close to Pluto, compared to other observed systems. Moons could potentially orbit Pluto at up to 53% (or 69%, if retrograde) of the Hill radius, the stable gravitational zone of Pluto's influence. For example, Psamathe orbits Neptune at 40% of the Hill radius. In the case of Pluto, only the inner 3% of the zone is known to be occupied by satellites. In the discoverers' terms, the Plutonian system appears to be "highly compact and largely empty",[107] although others have pointed out the possibility of additional objects, including a small ring system.[108][109]
Charon
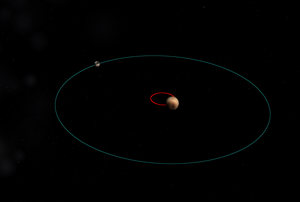
The Pluto–Charon system is noteworthy for being one of the Solar System's few binary systems, defined as those whose barycenter lies above the primary's surface (617 Patroclus is a smaller example, the Sun and Jupiter the only larger one).[110] This and the large size of Charon relative to Pluto has led some astronomers to call it a dwarf double planet.[111] The system is also unusual among planetary systems in that each is tidally locked to the other: Charon always presents the same face to Pluto, and Pluto always presents the same face to Charon: from any position on either body, the other is always at the same position in the sky, or always obscured.[112] This also means that the rotation period of each is equal to the time it takes the entire system to rotate around its common center of gravity.[68] Just as Pluto revolves on its side relative to the orbital plane, so the Pluto–Charon system does also.[69] In 2007, observations by the Gemini Observatory of patches of ammonia hydrates and water crystals on the surface of Charon suggested the presence of active cryo-geysers.[113]
Small moons
Two additional moons were imaged by astronomers working with the Hubble Space Telescope on May 15, 2005, and received provisional designations of S/2005 P 1 and S/2005 P 2. The International Astronomical Union officially named Pluto's newest moons Nix (or Pluto II, the inner of the two moons, formerly P 2) and Hydra (Pluto III, the outer moon, formerly P 1), on June 21, 2006.[114]
These small moons orbit Pluto at approximately two and three times the distance of Charon: Nix at 48,700 kilometres and Hydra at 64,800 kilometres from the barycenter of the system. They have nearly circular prograde orbits in the same orbital plane as Charon.
Observations of Nix and Hydra to determine individual characteristics are ongoing. Hydra is sometimes brighter than Nix, suggesting either that it is larger or that different parts of its surface may vary in brightness. Their sizes are estimated from albedos. If their albedo is similar to that of Charon (0.35), then their diameters are 46 kilometres for Nix and 61 kilometres for Hydra. Upper limits on their diameters can be estimated by using the albedo of the darkest Kuiper-belt objects (0.04); these bounds are 137 ± 11 km and 167 ± 10 km, respectively. At the larger end of this range, the inferred masses are less than 0.3% that of Charon, or 0.03% that of Pluto.[115]
The discovery of Nix and Hydra suggests that Pluto may possess a variable ring system. Small-body impacts can create debris that can form into planetary rings. Data from a deep-optical survey by the Advanced Camera for Surveys on the Hubble Space Telescope suggest that no ring system is present. If such a system exists, it is either tenuous like the rings of Jupiter or is tightly confined to less than 1,000 km in width.[108] Similar conclusions have been made from occultation studies.[116]
A fourth moon, Kerberos, was announced on July 20, 2011. It was detected using NASA's Hubble Space Telescope during a survey searching for rings around Pluto. It has an estimated diameter of 13 to 34 km and is located between the orbits of Nix and Hydra.[105] Kerberos was first seen in a photo taken with Hubble's Wide Field Camera 3 on June 28. It was confirmed in subsequent Hubble pictures taken on July 3 and July 18.[105]
A fifth moon, Styx, was announced on July 7, 2012 while looking for potential hazards for New Horizons.[117] Styx is believed to have a diameter of between 10 and 25 km and to orbit Pluto at a distance between Charon and Nix.[118]
Near resonances
Styx, Nix, Kerberos and Hydra are fairly close to 3:1, 4:1, 5:1 and 6:1 mean-motion orbital resonances with Charon, respectively[119][120] (the ratios approach integral commensurabilities more closely going outward from Pluto). Determining how near any of these orbital period ratios actually is to a true resonance requires accurate knowledge of the satellites' precessions.Name (Pronunciation) |
Discovery Year |
Diameter (km) |
Mass (kg) |
Orbital radius (km) (barycentric) |
Orbital period (d) | Period ratio | Magnitude (mag) | |
---|---|---|---|---|---|---|---|---|
Pluto | /ˈpluːtoʊ/ | 1930 | 2,306 (66% Moon) |
1.305×1022 (18% Moon) |
2,035 | 6.3872 (25% Moon) |
1.000 | 15.1 |
Charon | /ˈʃærən/, /ˈkɛərən/ |
1978 | 1,205 (35% Moon) |
1.52×1021 (2% Moon) |
17,536 (5% Moon) |
6.3872 (25% Moon) |
1.000 | 16.8 |
Styx | /ˈstɪks/ | 2012 | 10–25 | ? | ~42,000 ± 2,000 | 20.2 ± 0.1 | 3.16 | 27 |
Nix | /ˈnɪks/ | 2005 | 91 | 4×1017 | 48,708 | 24.856 | 3.892 | 23.7 |
Kerberos | /ˈkɛərbərəs/ | 2011 | 13–34 | ? | ~59,000 | 32.1 | 5.03 | 26 |
Hydra | /ˈhaɪdrə/ | 2005 | 114 | 8×1017 | 64,749 | 38.206 | 5.982 | 23.3 |
Quasi-satellite
At least one minor body is trapped in the 1:1 commensurability with Pluto, (15810) 1994 JR1, specifically in the quasi-satellite dynamical state.[122] The object has been a quasi-satellite of Pluto for about 100,000 years and it will remain in that dynamical state for perhaps another 250,000 years. Its quasi-satellite behavior is recurrent with a periodicity of 2 million years.[122][123] There may be additional Pluto co-orbitals.Origins
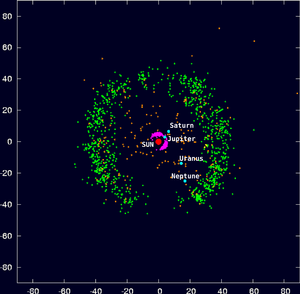
Plot of known Kuiper belt objects, set against the four gas giants.
Pluto's origin and identity had long puzzled astronomers. One early hypothesis was that Pluto was an escaped moon of Neptune, knocked out of orbit by its largest current moon, Triton. This notion has been heavily criticized because Pluto never comes near Neptune in its orbit.[124]
Pluto's true place in the Solar System began to reveal itself only in 1992, when astronomers began to find small icy objects beyond Neptune that were similar to Pluto not only in orbit but also in size and composition. This trans-Neptunian population is believed to be the source of many short-period comets. Astronomers now believe Pluto to be the largest[j] member of the Kuiper belt, a somewhat stable ring of objects located between 30 and 50 AU from the Sun. As of 2011, surveys of the Kuiper belt to magnitude 21 were nearly complete and any remaining Pluto-sized objects are expected to be beyond 100 AU from the Sun.[125] Like other Kuiper-belt objects (KBOs), Pluto shares features with comets; for example, the solar wind is gradually blowing Pluto's surface into space, in the manner of a comet.[126] If Pluto were placed as near to the Sun as Earth, it would develop a tail, as comets do.[127]
Though Pluto is the largest of the Kuiper belt objects discovered,[j] Neptune's moon Triton, which is slightly larger than Pluto, is similar to it both geologically and atmospherically, and is believed to be a captured Kuiper belt object.[128] Eris (see below) is about the same size as Pluto (though more massive) but is not strictly considered a member of the Kuiper belt population. Rather, it is considered a member of a linked population called the scattered disc.
A large number of Kuiper belt objects, like Pluto, possess a 2:3 orbital resonance with Neptune. KBOs with this orbital resonance are called "plutinos", after Pluto.[129]
Like other members of the Kuiper belt, Pluto is thought to be a residual planetesimal; a component of the original protoplanetary disc around the Sun that failed to fully coalesce into a full-fledged planet. Most astronomers agree that Pluto owes its current position to a sudden migration undergone by Neptune early in the Solar System's formation. As Neptune migrated outward, it approached the objects in the proto-Kuiper belt, setting one in orbit around itself (Triton), locking others into resonances, and knocking others into chaotic orbits. The objects in the scattered disc, a dynamically unstable region overlapping the Kuiper belt, are believed to have been placed in their current positions by interactions with Neptune's migrating resonances.[130] A computer model created in 2004 by Alessandro Morbidelli of the Observatoire de la Côte d'Azur in Nice suggested that the migration of Neptune into the Kuiper belt may have been triggered by the formation of a 1:2 resonance between Jupiter and Saturn, which created a gravitational push that propelled both Uranus and Neptune into higher orbits and caused them to switch places, ultimately doubling Neptune's distance from the Sun. The resultant expulsion of objects from the proto-Kuiper belt could also explain the Late Heavy Bombardment 600 million years after the Solar System's formation and the origin of the Jupiter trojans.[131] It is possible that Pluto had a near-circular orbit about 33 AU from the Sun before Neptune's migration perturbed it into a resonant capture.[132] The Nice model requires that there were about a thousand Pluto-sized bodies in the original planetesimal disk; these may have included the early Triton and Eris.[131]
Exploration

New Horizons, launched on January 19, 2006
Pluto presents significant challenges for spacecraft because of its small mass and large distance from Earth. Voyager 1 could have visited Pluto, but controllers opted instead for a close flyby of Saturn's moon Titan, resulting in a trajectory incompatible with a Pluto flyby. Voyager 2 never had a plausible trajectory for reaching Pluto.[133] No serious attempt to explore Pluto by spacecraft occurred until the last decade of the 20th century. In August 1992, JPL scientist Robert Staehle telephoned Pluto's discoverer, Clyde Tombaugh, requesting permission to visit his planet. "I told him he was welcome to it," Tombaugh later remembered, "though he's got to go one long, cold trip."[134] Despite this early momentum, in 2000, NASA cancelled the Pluto Kuiper Express mission, citing increasing costs and launch vehicle delays.[135]
After an intense political battle, a revised mission to Pluto, dubbed New Horizons, was granted funding from the US government in 2003.[136] New Horizons was launched successfully on January 19, 2006. The mission leader, S. Alan Stern, confirmed that some of the ashes of Clyde Tombaugh, who died in 1997, had been placed aboard the spacecraft.[137]
In early 2007 the craft made use of a gravity assist from Jupiter. Its closest approach to Pluto will be on July 14, 2015; scientific observations of Pluto will begin 5 months before closest approach and will continue for at least a month after the encounter. New Horizons captured its first (distant) images of Pluto in late September 2006, during a test of the Long Range Reconnaissance Imager (LORRI).[138] The images, taken from a distance of approximately 4.2 billion kilometres, confirm the spacecraft's ability to track distant targets, critical for maneuvering toward Pluto and other Kuiper Belt objects.
New Horizons will use a remote sensing package that includes imaging instruments and a radio science investigation tool, as well as spectroscopic and other experiments, to characterise the global geology and morphology of Pluto and its moon Charon, map their surface composition and analyse Pluto's neutral atmosphere and its escape rate. New Horizons will also photograph the surfaces of Pluto and Charon.
Pluto's small moons, discovered shortly before or after the probes's launch, may present it with unforeseen challenges. Debris from collisions between Kuiper belt objects and the smaller moons, with their relatively low escape velocities, may produce a tenuous dusty ring. Were New Horizons to fly through such a ring system, there would be an increased potential for micrometeoroid damage that could disable the probe.[108]
Concepts
A Pluto orbiter/lander/sample return mission was proposed in 2003. The plan included a twelve-year trip from Earth to Pluto, mapping from orbit, multiple landings, a warm water probe, and possible in situ propellant production for another twelve-year trip back to Earth with samples. Power and propulsion would come from the bimodal MITEE nuclear reactor system.[139]Classification
After Pluto's place within the Kuiper belt was determined, its official status as a planet became controversial, with many questioning whether Pluto should be considered together with or separately from its surrounding population.Museum and planetarium directors occasionally created controversy by omitting Pluto from planetary models of the Solar System. The Hayden Planetarium reopened after renovation in 2000 with a model of only eight planets. The controversy made headlines at the time.[140]
In 2002, the KBO 50000 Quaoar was discovered, with a diameter then thought to be roughly 1280 kilometres, about half that of Pluto.[141] In 2004, the discoverers of 90377 Sedna placed an upper limit of 1800 km on its diameter, nearer to Pluto's diameter of 2320 km,[142] although Sedna's diameter was revised downward to less than 1600 km by 2007.[143] Just as Ceres, Pallas, Juno and Vesta eventually lost their planet status after the discovery of many other asteroids, so, it was argued, Pluto should be reclassified as one of the Kuiper belt objects.
On July 29, 2005, the discovery of a new trans-Neptunian object was announced. Named Eris, it is now known to be approximately the same size as Pluto.[88] This was the largest object discovered in the Solar System since Triton in 1846. Its discoverers and the press initially called it the tenth planet, although there was no official consensus at the time on whether to call it a planet.[144] Others in the astronomical community considered the discovery the strongest argument for reclassifying Pluto as a minor planet.[145]
2006: IAU classification
The debate came to a head in 2006 with an IAU resolution that created an official definition for the term "planet". According to this resolution, there are three main conditions for an object to be considered a 'planet':- The object must be in orbit around the Sun.
- The object must be massive enough to be a sphere by its own gravitational force. More specifically, its own gravity should pull it into a shape of hydrostatic equilibrium.
- It must have cleared the neighborhood around its orbit.[146][147]
On September 13, 2006, the IAU included Pluto, Eris, and the Eridian moon Dysnomia in their Minor Planet Catalogue, giving them the official minor planet designations "(134340) Pluto", "(136199) Eris", and "(136199) Eris I Dysnomia".[149] If Pluto had been given a minor planet name upon its discovery, the number would have been about 1,164 rather than 134,340.
There has been some resistance within the astronomical community toward the reclassification.[150][151][152] S. Alan Stern, principal investigator with NASA's New Horizons mission to Pluto, publicly derided the IAU resolution, stating that "the definition stinks, for technical reasons".[153] Stern's contention was that by the terms of the new definition Earth, Mars, Jupiter, and Neptune, all of which share their orbits with asteroids, would be excluded.[154] His other claim was that because less than five percent of astronomers voted for it, the decision was not representative of the entire astronomical community.[154] Marc W. Buie, then at Lowell Observatory, voiced his opinion on the new definition on his website and petitioned against the definition.[155] Others have supported the IAU. Mike Brown, the astronomer who discovered Eris, said "through this whole crazy circus-like procedure, somehow the right answer was stumbled on. It's been a long time coming. Science is self-correcting eventually, even when strong emotions are involved."[156]
Researchers on both sides of the debate gathered on August 14–16, 2008, at the Johns Hopkins University Applied Physics Laboratory for a conference that included back-to-back talks on the current IAU definition of a planet.[157] Entitled "The Great Planet Debate",[158] the conference published a post-conference press release indicating that scientists could not come to a consensus about the definition of planet.[159] Just before the conference, on June 11, 2008, the IAU announced in a press release that the term "plutoid" would henceforth be used to describe Pluto and other objects similar to Pluto which have an orbital semimajor axis greater than that of Neptune and enough mass to be of near-spherical shape.[148][160][161]
Reaction
Reception to the IAU decision was mixed. Although many accepted the reclassification, some sought to overturn the decision with online petitions urging the IAU to consider reinstatement. A resolution introduced by some members of the California State Assembly light-heartedly denounced the IAU for "scientific heresy", among other crimes.[162] The U.S. state of New Mexico's House of Representatives passed a resolution in honor of Tombaugh, a longtime resident of that state, which declared that Pluto will always be considered a planet while in New Mexican skies and that March 13, 2007, was Pluto Planet Day.[163][164] The Illinois State Senate passed a similar resolution in 2009, on the basis that Clyde Tombaugh, the discoverer of Pluto, was born in Illinois. The resolution asserted that Pluto was "unfairly downgraded to a 'dwarf' planet" by the IAU.[165]
Some members of the public have also rejected the change, citing the disagreement within the scientific community on the issue, or for sentimental reasons, maintaining that they have always known Pluto as a planet and will continue to do so regardless of the IAU decision.[166]
In 2006 in its 17th annual words of the year vote, the American Dialect Society voted plutoed as the word of the year. To "pluto" is to "demote or devalue someone or something".[167]