New Horizons
Artist's concept of New Horizons when it reaches Pluto in July 2015
|
|
Mission type |
Pluto flyby |
Operator |
NASA |
COSPAR ID |
2006-001A |
SATCAT № |
28928 |
Website |
pluto.jhuapl.edu |
Mission duration |
Primary mission: 9.5 years |
|
Spacecraft properties |
Manufacturer |
APL · Southwest Research Institute |
Launch mass |
478 kilograms (1,054 lb) |
Power |
228 watts |
|
Start of mission |
Launch date |
January 19, 2006 (2006-01-19) 19:00 UTC
(9 years, 1 month and 9 days ago) |
Rocket |
Atlas V 551 |
Launch site |
Space Launch Complex 41
Cape Canaveral Air Force Station, Florida, United States |
Contractor |
International Launch Services |
|
Flyby of 7005132524000000000(132524) APL (incidental) |
Closest approach |
June 13, 2006 (2006-06-13) 04:05 UTC
(8 years, 8 months and 15 days ago) |
Distance |
101,867 km (63,297 mi) |
Flyby of Jupiter (Gravity assist) |
Closest approach |
February 28, 2007 (2007-02-28) 05:43:40 UTC
(8 years ago) |
Distance |
2,300,000 km (1,400,000 mi) |
Flyby of Pluto |
Closest approach |
July 14, 2015 (2015-07-14) 11:49:59 UTC
(165 days to go) |
Distance |
12,500 km (7,800 mi) |
[show]Instruments |
Alice |
Ultraviolet Imaging Spectrometer |
LORRI |
Long Range Reconnaissance Imager |
SWAP |
Solar Wind at Pluto |
PEPSSI |
Pluto Energetic Particle Spectrometer Science Investigation |
REX |
Radio Science Experiment |
Ralph |
Ralph Telescope |
SDC |
Venetia Burney Student Dust Counter |
|
 |
New Horizons is a
NASA space probe launched to study the
dwarf planet Pluto, its
moons and one or two other
Kuiper belt objects, depending on which are in position to be explored. Part of the
New Frontiers program, the mission was approved in 2001 after cancellation of
Pluto Fast Flyby and
Pluto Kuiper Express. The mission profile was proposed by a team led by principal investigator
Alan Stern of
Southwest Research Institute. After several delays on the launch site,
New Horizons was launched on January 19, 2006 from
Cape Canaveral, directly into an
Earth-and-solar-escape trajectory with an Earth-relative speed of about 16.26 kilometers per second (58,536 km/h; 36,373 mph); it set the record for the highest launch speed of a human-made object from
Earth. After a brief encounter with asteroid
132524 APL,
New Horizons proceeded to
Jupiter, making its closest approach on February 28, 2007 at a distance of 2.3 million kilometers (1.4 million miles). The Jupiter flyby provided a
gravity assist that increased
New Horizons ' speed by 4 km/s (14,000 km/h; 9,000 mph). The encounter was also used as a general test of
New Horizons ' scientific capabilities, returning data about the planet's
atmosphere,
moons and
magnetosphere. After Jupiter, the probe continued towards Pluto. Much of the post-Jupiter voyage has been spent in hibernation mode to preserve onboard systems. On January 15, 2015, NASA reported the New Horizons spacecraft began its approach phase to Pluto, which will result in the first
flyby of the dwarf planet on July 14, 2015.
[1]
Background
New Horizons on the launchpad
New Horizons is the first mission in NASA's
New Frontiers mission category, larger and more expensive than the
Discovery missions but smaller than the
Flagship Program. The cost of the mission (including spacecraft and instrument development, launch vehicle, mission operations, data analysis, and education/public outreach) is approximately $650 million over 15 years (2001–2016). An earlier proposed Pluto mission—
Pluto Kuiper Express—was cancelled by NASA in 2000 for budgetary reasons. After a three month concept study, NASA announced on June 8, 2001 that of the two competing design proposals,
New Horizons and POSSE (Pluto and Outer Solar System Explorer),
New Horizons will proceed with preliminary design studies for a Pluto flyby mission.
[2]
The spacecraft was built primarily by
Southwest Research Institute (SwRI) and the
Johns Hopkins Applied Physics Laboratory. The mission's
principal investigator is
Alan Stern of the Southwest Research Institute (formerly NASA Associate Administrator).
Overall control after separation from the launch vehicle is performed at Mission Operations Center (MOC) at the
Applied Physics Laboratory. The science instruments are operated at Clyde Tombaugh Science Operations Center (T-SOC) in
Boulder, Colorado.
[3] Navigation, which is not real-time, is performed at various contractor facilities, while the navigational positional data and related celestial reference frames are provided by the
Naval Observatory Flagstaff Station through Headquarters NASA and JPL;
KinetX is the lead on the
New Horizons navigation team and is responsible for planning trajectory adjustments as the spacecraft speeds toward
the outer Solar System.
New Horizons was originally planned as a voyage to the only unexplored planet in the Solar
System. When the spacecraft was launched, Pluto was still classified as a
planet, later to be
reclassified as a dwarf planet by the
International Astronomical Union (IAU). Some members of the
New Horizons team, including Alan Stern, disagree with the IAU definition and still describe Pluto as the ninth planet.
[4] Pluto's satellites
Nix and
Hydra also have a connection with the spacecraft: the first letters of their names (N and H) are the initials of
New Horizons. The moons' discoverers chose these names for this reason, plus Nix and Hydra's relationship to the mythological
Pluto.
[5]
In addition to the science equipment, there are several cultural artifacts traveling with the spacecraft. These include a collection of 434,738 names stored on a compact disc,
[6] a piece of Scaled Composites
SpaceShipOne,
[7] and a
flag of the USA, along with other mementos.
[8]
About an ounce of
Clyde Tombaugh's ashes are aboard the spacecraft, to commemorate his discovery of Pluto in 1930.
[9][10] A Florida-
state quarter coin, whose design commemorates human exploration, is included, officially as a trim weight.
[11] One of the science packages (a dust counter) is named after
Venetia Burney, who, as a child, suggested the name "Pluto" after the planet's discovery.
Design and construction
Spacecraft subsystems
The spacecraft is comparable in size and general shape to a
grand piano and has been compared to a piano glued to a cocktail bar-sized satellite dish.
[12] As a point of departure, the team took inspiration from the
Ulysses spacecraft,
[13] which also carried a
radioisotope thermoelectric generator (RTG) and dish on a box-in-box structure through the outer Solar System. Many subsystems and components have flight heritage from APL's
CONTOUR spacecraft, which in turn had heritage from APL's
TIMED spacecraft.
The spacecraft's body forms a triangle, almost 0.76 m (2.5 ft) thick. (The
Pioneers have
hexagonal bodies, while the
Voyagers,
Galileo, and
Cassini–Huygens have
decagonal, hollow bodies.) A
7075 aluminium alloy tube forms the main structural column, between the launch vehicle adapter ring at the "rear," and the 2.1 m (6 ft 11 in) radio
dish antenna affixed to the "front" flat side. The
titanium fuel tank is in this tube. The RTG attaches with a 4-sided titanium mount resembling a grey pyramid or stepstool. Titanium provides strength and thermal isolation. The rest of the triangle is primarily sandwich panels of thin aluminium facesheet (less than
1⁄64 in or 0.40 mm) bonded to aluminium honeycomb core. The structure is larger than strictly necessary, with empty space inside. The structure is designed to act as
shielding, reducing electronics
errors caused by radiation from the RTG. Also, the mass distribution required for a spinning spacecraft demands a wider triangle.
Internally, the structure is painted black. This equalizes temperature by
radiative heat transfer. Overall, the spacecraft is thoroughly blanketed to retain heat. Unlike the Pioneers and Voyagers, the radio dish is also enclosed in blankets which extend to the body. The heat from the RTG also adds warmth to the spacecraft in the outer Solar System. In the inner Solar System, the spacecraft must prevent overheating. Electronic activity is limited, power is diverted to
shunts with attached radiators, and
louvers are opened to radiate excess heat. Then, when the spacecraft is cruising inactively in the cold outer Solar System, the louvers are closed, and the shunt regulator reroutes power to electric
heaters.
Propulsion and attitude control
New Horizons has both spin-stabilized (cruise) and three-axis stabilized (science) modes controlled entirely with
hydrazine monopropellant. Additional post launch
delta-v of over 290 m/s (1,000 km/h; 650 mph) is provided by a 77 kg (170 lb) internal tank. Helium is used as a pressurant, with an
elastomeric diaphragm assisting expulsion. The spacecraft's on-orbit mass including fuel is over 470 kg (1,040 lb) on the Jupiter flyby trajectory, but would have been only 445 kg (981 lb) for the backup direct flight option to Pluto. Significantly, had the backup option been taken, this would have meant less fuel for later Kuiper belt operations.
There are 16
thrusters on
New Horizons: four 4.4
N (1.0
lbf) and twelve 0.9 N (0.2 lbf) plumbed into redundant branches. The larger thrusters are used primarily for trajectory corrections, and the small ones (previously used on
Cassini and the
Voyager spacecraft) are used primarily for
attitude control and spinup/spindown maneuvers. Two star cameras (from Galileo Avionica) are used for fine attitude control. They are mounted on the face of the spacecraft and provide attitude information while in spin-stabilized or 3-axis mode. Between star camera readings, knowledge is provided by dual redundant
Miniature Inertial Measurement Unit (MIMU) from
Honeywell. Each unit contains three solid-state gyroscopes and three accelerometers. Two
Adcole Sun sensors provide attitude control. One detects angle to the Sun while the other measures spin rate and clocking.
Power
New Horizons ' radioisotope thermoelectric generator (RTG) at the Kennedy Space Center’s Payload Hazardous Servicing Facility
A cylindrical
radioisotope thermoelectric generator (RTG), protrudes from one vertex in the plane of the triangle. The RTG will provide about
250 W, 30 V DC at launch, and is predicted to drop approximately 5% every 4
years, decaying to
200 W by the encounter with the
Plutonian system in 2015. The RTG, model "
GPHS-RTG," was originally a spare from the
Cassini mission. The RTG contains 11 kg (24 lb) of
plutonium-238 oxide pellets. Each pellet is clad in
iridium, then encased in a graphite shell. It was developed by the U.S.
Department of Energy at the Materials and Fuels Complex (formerly Argonne West), a part of the
Idaho National Laboratory in
Bingham County, near the town of
Arco and the city of
Idaho Falls.
[14] Less than the original design goal was produced because of delays at the United States Department of Energy, including security activities, that held up production. The mission parameters and observation sequence had to be modified for the reduced wattage; still, not all instruments can operate simultaneously. The Department of Energy transferred the space battery program from Ohio to Argonne in 2002 because of security concerns. There are no onboard batteries. RTG output is relatively predictable; load transients are handled by a capacitor bank and fast circuit breakers.
The amount of radioactive plutonium in the RTG is 10.9 kg (24 lb), about one-third the amount on board the
Cassini–Huygens probe when it launched in 1997. That launch was protested by some people. The
United States Department of Energy estimated the chances of a launch accident that would release radiation into the atmosphere at 1 in 350, and monitored the launch
[15] as it always does when RTGs are involved. It was believed that a worst-case scenario of total dispersal of on-board plutonium would spread the equivalent radiation of 80% the average annual dosage in North America from background radiation over an area with a radius of 105 km (65 mi).
[16]
Telecommunications and data handling
Communication with the spacecraft is via
X band. The craft had a communication rate of
38 kbit/s at Jupiter; at Pluto's distance, a rate of approximately
1 kbit/s is expected. Besides the low bandwidth, Pluto's distance also causes a (one-way)
latency of about 4.5
hours. The 70 m (230 ft)
Deep Space Network (DSN) dishes are used to relay data beyond Jupiter. The spacecraft uses dual redundant transmitters and receivers, and either right- or left-hand circular
polarization. The downlink signal is amplified by dual redundant 12-watt
traveling-wave tube amplifiers (TWTAs) mounted on the body under the dish. The receivers are new, low-power designs. The system can be controlled to power both TWTAs at the same time, and transmit a dual-polarized downlink signal to the DSN that could almost double the downlink rate. Initial tests with the DSN in this dual-polarized mode have been successful, and an effort to make the DSN polarization-combining technique operational is underway.
Antennas of
New Horizons (HGA, MGA and LGA)
In addition to the high-gain antenna, there are two low-gain antennas and a medium-gain dish. The high-gain dish has a
Cassegrain layout, composite construction, and a 2.1-meter (7 ft) diameter (providing well over
40 decibel of gain, and a half-power beam width of about a degree). The prime-focus, medium-gain antenna, with a 0.3-meter (1 ft) aperture and 10° half-power beamwidth, is mounted to the back of the high-gain antenna's secondary reflector. The forward low-gain antenna is stacked atop the feed of the medium-gain antenna. The aft low-gain antenna is mounted within the launch adapter at the rear of the spacecraft. This antenna was used only for early mission phases near Earth, just after launch and for emergencies if the spacecraft had lost
attitude control.
New Horizons will record scientific instrument data to its solid-state buffer at each encounter, then transmit the data to Earth. Data storage is done on two low-power
solid-state recorders (one primary, one backup) holding up to
8 gigabytes each. Because of the extreme distance from Pluto and the Kuiper belt, only one buffer load at those encounters can be saved. This is because
New Horizons will have left the vicinity of Pluto (or future target object) by the time it takes to transmit the buffer load back to Earth.
Part of the reason for the delay between the gathering and transmission of data is because all of the
New Horizons instrumentation is body-mounted. In order for the cameras to record data, the entire probe must turn, and the one-degree-wide beam of the high-gain antenna will almost certainly not be pointing toward Earth. Previous spacecraft, such as the
Voyager program probes, had a rotatable instrumentation platform (a "scan platform") that could take measurements from virtually any angle without losing radio contact with Earth.
New Horizons ' elimination of excess mechanisms was implemented to save weight, shorten the schedule, and improve reliability to achieve a 15-year lifetime.
(The
Voyager 2 spacecraft experienced platform jamming at Saturn; the demands of long time exposures at Uranus led to modifications of the mission such that the entire probe was rotated to achieve the time exposure photos at Uranus and Neptune, similar to how
New Horizons will rotate.)
Flight computer
The spacecraft carries two
computer systems, the Command and Data Handling system and the Guidance and Control processor. Each of the two systems is duplicated for
redundancy, for a total of four computers. The processor used is the
Mongoose-V, a 12
MHz radiation-hardened version of the
MIPS R3000 CPU. Multiple clocks and timing routines are implemented in hardware and software to help prevent faults and downtime.
To conserve heat and mass, spacecraft and instrument electronics are housed together in IEMs (Integrated Electronics Modules). There are two redundant IEMs. Including other functions such as instrument and radio electronics, each IEM contains 9
boards.
On March 19, 2007 the Command and Data Handling computer experienced an uncorrectable memory error and rebooted itself, causing the spacecraft to go into
safe mode. The craft fully recovered within two days, with some data loss on Jupiter's
magnetotail. No impact on the subsequent mission is expected.
[17]
Mission science
New Horizons carries seven instruments: three optical instruments, two plasma instruments, a dust sensor and a radio science receiver/radiometer. The instruments are to be used to investigate the global geology, surface composition and temperature, and the atmospheric pressure, temperature and escape rate of Pluto and its moons. The total mass of the spacecraft is 31 kg (68 lb) and rated power is
21 watts (though not all instruments operate simultaneously).
[18] In addition,
New Horizons has an Ultrastable Oscillator subsystem, which may be used to study and test the
Pioneer Anomaly towards the end of the spacecraft's life.
[19]
Alice |
|
Alice is an ultraviolet imaging spectrometer that makes one of two photographic instruments comprising New Horizons ' Pluto Exploration Remote Sensing Investigation (PERSI). The other being the Ralph telescope. It resolves 1,024 wavelength bands in the far and extreme ultraviolet (from 50–180 nm), over 32 view fields. Its goal is to view the atmospheric makeup of Pluto. This Alice instrument is derived from an another Alice aboard the ESA's Rosetta spacecraft.
|
Long Range Reconnaissance Imager (LORRI) |
 |
LORRI is a long focal length imager designed for high resolution and responsivity at visible wavelengths. The instrument is equipped with a high-resolution 1024×1024 monochromatic CCD imager with a 208.3 mm (8.20 in) aperture giving a resolution of 5 μrad (~1 asec). The CCD is chilled far below freezing by a passive radiator on the antisolar face of the spacecraft. This temperature differential requires insulation, and isolation from the rest of the structure. The Ritchey–Chretien mirrors and metering structure are made of silicon carbide, to boost stiffness, reduce weight, and prevent warping at low temperatures. The optical elements sit in a composite light shield, and mount with titanium and fibreglass for thermal isolation. Overall mass is 8.6 kg (19 lb), with the Optical tube assembly (OTA) weighing about 5.6 kg (12 lb),[20] for one of the largest silicon-carbide telescopes flown at the time (now superseded by Herschel).
|
Solar Wind at Pluto (SWAP) |
 |
SWAP (Solar Wind At Pluto) is a toroidal electrostatic analyzer and retarding potential analyzer, that makes up one of the two instruments comprising New Horizons ' Plasma and high-energy particle spectrometer suite (PAM). The other being PEPSSI. SWAP measures particles of up to 6.5 keV and, because of the tenuous solar wind at Pluto's distance, the instrument has the largest aperture of any such instrument ever flown.
|
Pluto Energetic Particle Spectrometer Science Investigation (PEPSSI) |
|
PEPSSI is a time of flight ion and electron sensor that makes up one of the two instruments comprising New Horizons ' Plasma and high-energy particle spectrometer suite (PAM). The other being SWAP. Unlike SWAP, which measures particles of up to 6.5 keV, PEPSSI goes up to 1 MeV.
|
Radio Science Experiment (REX) |
|
REX will use an ultrastable crystal oscillator (essentially a calibrated crystal in a miniature oven) and some additional electronics to conduct radio science investigations using the communications channels. These are small enough to fit on a single card. Since there are two redundant communications subsystems, there are two, identical REX circuit boards. There is an outstanding request for a series of Geophysical Telegrams to be issued so that the REX can avoid failures and obtain more scientifically useful information.[1]
-
REX information, from a BOINC screensaver
-
BOINC screensaver, Charon Radio Science
-
REX information, link to PDF of Radio Science Proposal
|
Ralph |
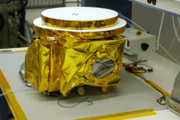 |
The Ralph telescope, 6 cm (2.4 in) in aperture, is one of two photographic instruments that make up New Horizons ' Pluto Exploration Remote Sensing Investigation (PERSI), with the other being the Alice instrument. Ralph has two separate channels: a visible-light CCD imager (MVIC- Multispectral Visible Imaging Camera) with broadband and color channels, and a near-infrared imaging spectrometer, LEISA (Linear Etalon Imaging Spectral Array). LEISA is derived from a similar instrument on the EO-1 mission. Ralph was named after Alice's husband on The Honeymooners, and was designed after Alice.
|
Venetia Burney Student Dust Counter (SDC) |
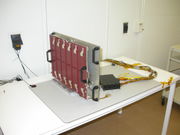 |
Built by students at the University of Colorado Boulder, the Student Dust Counter will operate continuously through the trajectory to make dust measurements. It consists of a detector panel, about 460 mm × 300 mm (18 in × 12 in), mounted on the antisolar face of the spacecraft (the ram direction), and an electronics box within the spacecraft. The detector contains fourteen polyvinylidene difluoride (PVDF) panels, twelve science and two reference, which generate voltage when impacted. Effective collecting area is 0.125 m2 (1.35 sq ft). No dust counter has operated past the orbit of Uranus; models of dust in the outer Solar System, especially the Kuiper belt, are speculative. VBSDC is always turned on measuring the masses of the interplanetary and interstellar dust particles (in the range of nano- and picograms) as they collide with the PVDF panels mounted on the New Horizons spacecraft. The measured data is expected to greatly contribute to the understanding of the dust spectra of the Solar System. The dust spectra can then be compared with those observed via telescope of other stars, giving new clues as to where earthlike planets can be found in our universe. The dust counter is named for Venetia Burney, who first suggested the name "Pluto" at the age of 11. A thirteen-minute short film about VBSDC garnered an Emmy award for student achievement in 2006.[21]
|
Launch
The
Atlas V rocket carrying
New Horizons lifts off from Cape Canaveral. The launch was the fastest ever to date, at 16.26km/s.
On September 24, 2005 the spacecraft arrived at the Kennedy Space Center on board a
C-17 Globemaster III for launch preparations.
[23] The launch of
New Horizons was originally scheduled for January 11, 2006, but was initially delayed until January 17, 2006 to allow for
borescope inspections of the
Atlas V's
kerosene tank. Further delays related to low cloud ceiling conditions
downrange, and high winds and technical difficulties—unrelated to the rocket itself—prevented launch for a further two days.
[24][25] Although there were backup launch opportunities in February 2006 and February 2007, only the first twenty-three days of the 2006 window permitted the Jupiter fly-by. Any launch outside that period would have forced the spacecraft to fly a slower trajectory directly to Pluto, delaying its encounter by 2–4 years. The probe finally lifted off from
Pad 41 at
Cape Canaveral Air Force Station,
Florida, directly south of
Space Shuttle Launch Complex 39, at 14:00 EST on Jan 19, 2006.
[26][27]
The
Centaur second stage reignited at 14:30 EST (19:30 UTC), followed by the
ATK Star 48B third stage, successfully sending the probe on a solar-escape trajectory.
New Horizons took only nine hours to reach the Moon's orbit, passing lunar orbit before midnight EST that day.
The probe was launched by a
Lockheed Martin Atlas V 551 rocket, with a third stage added to increase the heliocentric (escape) speed. This was the first launch of the Atlas V 551 configuration, which uses five
solid rocket boosters, and the first Atlas V with a third stage. Previous flights had used none, two, or three solid boosters, but never five. The vehicle, AV-010, weighed 570,000 kg (1.26 million lb) at lift-off, and had earlier been slightly damaged when
Hurricane Wilma swept across
Florida on October 24, 2005. One of the solid rocket boosters was hit by a door. The booster was replaced with an identical unit, rather than inspecting and requalifying the original.
[55]
New Horizons is often given the title of
Fastest Spacecraft Ever Launched, and left Earth faster than any other spacecraft to date. It is also the first spacecraft launched directly into a solar escape trajectory, which requires an approximate speed of 16.5 km/s (59,000 km/h; 37,000 mph), plus losses, all to be provided by the launcher. But it is not the fastest spacecraft to leave the Solar System. This record is held by
Voyager 1, currently travelling at 17.145 km/s (61,720 km/h; 38,350 mph) relative to the Sun.
Voyager 1 attained greater
hyperbolic excess velocity from Jupiter and Saturn
gravitational slingshots than
New Horizons. Other spacecraft, such as the
Helios probes, can also be measured as the fastest objects, due to their orbital speed relative to the Sun at
perihelion. Because they remain in solar orbit, their
specific orbital energy relative to the Sun is lower than
New Horizons and other
artificial objects escaping the Solar System.
The Star 48B third-stage is also on a
hyperbolic Solar System escape trajectory, and reached Jupiter before the
New Horizons spacecraft. Since it is not in controlled flight, it did not receive the correct gravity assist, and will only pass within 200 million km (120 million mi) of Pluto.
[56] The Centaur stage did not achieve solar escape velocity, and is in heliocentric orbit.
[57]
The launch was dedicated to the memory of launch conductor
Daniel Sarokon, who was described by space program officials as one of the most influential people in the history of space travel.
[58]
|
The Atlas V 551 rocket, used to launch New Horizons, being processed a month before launch.
|
|
Distant view of Cape Canaveral during the launch of New Horizons on January 19, 2006.
|
|
NASA TV footage of New Horizons ' launch from Cape Canaveral. (4:00)
|
|
Trajectory corrections and 132524 APL
Double image of the 2.5 km (1.6 mi) diameter asteroid
132524 APL, photographed by
New Horizons at two different distances
On January 28 and 30, 2006, mission controllers guided the probe through its first
trajectory correction maneuver (TCM), which was divided into two parts (TCM-1A and TCM-1B). The total velocity change of these two corrections was about 18 meters per second (65 km/h; 40 mph). TCM-1 was accurate enough to permit the cancellation of TCM-2, the second of three originally scheduled corrections.
[59]
During the week of February 20, 2006, controllers conducted initial in-flight tests of three onboard science instruments, the Alice ultraviolet imaging spectrometer, the PEPSSI plasma-sensor, and the LORRI long-range visible-spectrum camera. No scientific measurements or images were taken, but instrument electronics, and in the case of Alice, some electromechanical systems were shown to be functioning correctly.
[60]
On March 9, 2006 at 17:00 UTC, controllers performed TCM-3, the last of three scheduled course corrections. The engines burned for 76 seconds, adjusting the spacecraft's velocity by about 1.16 m/s (4.2 km/h; 2.6 mph).
[61]
On April 7, 2006 at 10:00 UTC, the spacecraft passed the orbit of Mars, moving at roughly 21 km/s (76,000 km/h; 47,000 mph) away from the Sun at a solar distance of 243 million kilometers.
[28][62][63]
Because of the need to conserve fuel for possible encounters with Kuiper belt objects subsequent to the Pluto flyby, intentional encounters with objects in the
asteroid belt were not planned. After launch, the
New Horizons team scanned the spacecraft's trajectory to determine if any asteroids would, by chance, be close enough for observation. In May
2006 it was discovered that
New Horizons would pass close to the tiny asteroid
132524 APL on June 13, 2006. Closest approach occurred at 4:05 UTC at a distance of 101,867 km (63,297 mi). The asteroid was imaged by Ralph (use of LORRI was not possible due to proximity to Sun), which gave the team a chance to exercise Ralph's capabilities, and make observations of the asteroid's composition as well as light and phase curves. The asteroid was estimated to be 2.5 km (1.6 mi) in diameter.
[64][65][66] The spacecraft successfully tracked the asteroid over June 10–12, 2006. This allowed the mission team to test the spacecraft's ability to track rapidly moving objects. Images were obtained through the Ralph telescope.
[66] On September 25, 2007 at 16:04 EDT, the engines were fired for 15 minutes and 37 seconds, changing the spacecraft's velocity by 2.37 m/s (8.5 km/h; 5.3 mph).
[67] On June 30, 2010 on 7:49 EDT, mission controllers executed a fourth TCM on
New Horizons that lasted 35.6 seconds.
[68]
Jupiter encounter
An
infrared image of Jupiter's southern atmosphere (
New Horizons;
LEISA).
New Horizons used LORRI to take its first photographs of Jupiter on September 4, 2006 from a distance of 291 million kilometers (181 million miles).
[69] More detailed exploration of the system began in January 2007 with an infrared image of the moon Callisto as well as several black and white images of the planet itself.
[70] New Horizons received a Jupiter
gravity assist with a closest approach at 05:43:40 UTC on February 28, 2007 when it was 2.3 million kilometers (1.4 million miles) from the planet. The flyby increased
New Horizons ' speed by 4 km/s (14,000 km/h; 9,000 mph) accelerating the probe 23 km/s (83,000 km/h; 51,000 mph) relative to the Sun and shortening its voyage to Pluto by three years.
[71]
The flyby was the center of a 4-month intensive observation campaign lasting from January to June. Being an ever-changing scientific target, Jupiter was observed intermittently since the end of the
Galileo mission. Knowledge about the planet benefited from the fact that
New Horizons instruments were built using the latest technology, especially in the area of cameras, representing a significant improvement over Galileo's cameras, which were evolved versions of
Voyager cameras which, in turn, were evolved
Mariner cameras. The Jupiter encounter also served as a shakedown and dress rehearsal for the Pluto encounter. Because of the much shorter distance from Jupiter to Earth, the communications link can transmit multiple loadings of the memory buffer; thus the mission actually returned more data from the Jovian system than it is expected to transmit from Pluto.
[72]
One of the main goals during the Jupiter encounter was observing the planet's
atmospheric conditions and analyzing the structure and composition of its clouds. Heat induced lightning strikes in the polar regions and "waves" that indicate violent storm activity were observed and measured. The
Little Red Spot, spanning up to 70% of Earth's diameter, was imaged from up close for the first time.
[71]
Observing from different angles and illumination conditions
New Horizons took detailed images of Jupiter's faint
ring system discovering debris left over from recent collisions within the rings or from some other unexplained phenomena. The search for undiscovered moons within the rings showed no results. Travelling through the planet's
magnetosphere New Horizons collected valuable particle readings.
[71] "Bubbles" of plasma which are believed to be formed from material ejected by the moon Io were noticed in the
magnetotail.
[73]
|
A LORRI image of Jupiter, taken on January 8, 2007, while the probe was 81 million kilometers from the planet and one month away from closest approach.
|
|
A composite image of Jupiter and Io, taken on on February 28 and March 1, 2007 respectively. Jupiter is shown in infrared, while Io is shown in true-color.
|
|
LORRI images of Jupiter's rings. The top image was taken on approach to Jupiter, while the bottom image was taken on departure, illuminated by the Sun.
|
|
A composite false-color image of Oval BA, otherwise known as the "Little Red Spot", using New Horizons ' LORRI and the Hubble Space Telescope 's WFPC2.
|
|
An MVIC image of Jupiter's equatorial atmosphere, showing Buoyancy waves which travel at 100m/s faster than surrounding clouds.
|
|
Jovian moons
The major (
Galilean) moons were in poor position; the aim of the gravity-assist maneuver meant the spacecraft passed millions of kilometers from any of the Galilean moons. Still, the
New Horizons instruments were intended for small, dim targets, so they were scientifically useful on large, distant moons. Emphasis was put on Io, whose active volcanoes shoot out tons of material into the planetary magnetosphere, and further. Out of 11 observed eruptions, three were seen for the first time while that of the volcano
Tvashtar rose up to an altitude of 330 kilometers. The event gave scientists an unprecedented look into the structure and motion of the rising plume and its subsequent fall back to the surface. Infrared signatures of a further 36 volcanoes were noticed.
[71] Callisto's surface was analyzed with LEISA, revealing how lighting and viewing conditions affect infrared spectrum readings of its surface water ice.
[74] Minor moons such as
Amalthea had their orbit solutions refined. The cameras determined their position, acting as "reverse optical navigation".
|
New Horizons view of Io. The Tvashtar volcano, which can be seen in the top of the image, had erupted during the spacecraft's flyby.
|
|
New Horizons view of Europa. One of the goals of New Horizons at Europa was to examine "nature of the icy crust and the forces that have deformed it".
|
|
New Horizons view of Callisto. The moon was used by the New Horizons team to practice detecting water ice, in preparation for similar experiments at Charon.
|
|
Transit (Jupiter to Pluto)
The first images of Pluto from
New Horizons were acquired September 21–24, 2006, during a test of the LORRI. They were released on November 28, 2006.
[31] The images, taken from a distance of approximately 4,200,000,000 km (2.6
×10
9 mi), confirmed the spacecraft's ability to track distant targets, critical for maneuvering toward Pluto and other
Kuiper belt objects.
|
September 2006: New Horizons takes first images of Pluto.
|
|
After passing Jupiter,
New Horizons spent most of its journey towards Pluto in hibernation mode: redundant components as well as guidance and control systems were shut down to extend their life cycle, decrease operation costs and free the
Deep Space Network for other missions.
[75] During hibernation mode, the onboard computer monitored the probe's systems and transmitted a signal back to Earth: a "green" code if everything was functioning as expected or a "red" code if the mission control's assistance were needed.
[75] The probe was activated for about two months a year so that the instruments could be calibrated and the systems checked. The first hibernation mode cycle started on June 28, 2007,
[75] the second cycle began on December 16, 2008,
[76] the third cycle on August 27, 2009,
[77] and the fourth cycle on August 29, 2014 after a 10 week test.
[78]
New Horizons crossed the orbit of
Saturn on June 8, 2008,
[79] and Uranus on March 18, 2011.
[80] After astronomers announced the discovery of two new moons in the Pluto system,
Kerberos and
Styx, mission planners started contemplating the possibility of the probe running into unseen debris and dust left over from earlier collisions with the moons. A study based on 18 months of computer simulations, Earth-based telescope observations and occultations of the Pluto system revealed that the possibility of a catastrophic collision with debris or dust is less than 0.3% on the probe's scheduled course.
[81][82] If the hazard increases,
New Horizons will use one of two possible contingency plans, the so-called SHBOTs (Safe Haven by Other Trajectories): the probe could continue on its present trajectory with the antenna facing the incoming particles so the more vital systems would be protected, or, it could position its antenna and make a course correction that would take it just 3000 km from the surface of Pluto where it is expected that the
atmospheric drag would clean the surrounding space of possible debris.
[82]
While in hibernation mode in July 2012,
New Horizons started gathering scientific data with SWAP, PEPSSI and SDC. Although it was originally planned to activate just SDC, other instruments were powered on the initiative of principal investigator Alan Stern who believed they could use the opportunity to collect valuable heliospheric data. Before activating the other two instruments, ground tests were conducted to make sure that the expanded data gathering in this phase of the mission would not limit available energy, memory and fuel in the future and that all systems are functioning during the flyby.
[83] The first set of data was transmitted in January 2013 during a three week activation from hibernation. A new command and data handling software was also uploaded to address the problem of computer resets.
[84]
Images from July 1 to 3, 2013 by LORRI were the first by the probe to resolve Pluto and Charon as separate objects.
[85]
On July 14, 2014, mission controllers performed a sixth trajectory correction maneuver (TCM) since its launch to enable the craft to reach Pluto.
[86]
Between July 19–24, 2014,
New Horizons ' LORRI snapped 12 images of Charon revolving around Pluto, covering almost one full rotation at distances ranging from about 429,000,000 km (267,000,000 mi) to 422,000,000 km (262,000,000 mi).
[87]
|
July 2014: New Horizons takes images of Pluto and its largest moon, Charon.
|
|
In August 2014, astronomers made high-precision measurements of Pluto's location and orbit around the Sun using the Atacama Large Millimeter/submillimeter Array (
ALMA) to help NASA’s
New Horizons spacecraft accurately hone in on Pluto.
[88]
|
August 2014: The ALMA observes Pluto and Charon.
|
|
On December 6, 2014, mission controllers sent a signal for the craft to "wake up" from its final Pluto-approach hibernation and begin regular operations. The craft's response that, it was "awake", arrived to Earth at 9:30 PM EST.
[49][89]
Pluto encounter
Distance-encounter operations at Pluto began on January 4, 2015.
[90] At this date images of the targets with the onboard LORRI imager plus Ralph telescope would only be a few
pixels in width. Investigators have begun taking Pluto and background starfield images to assist mission navigators in the design of course-correcting engine maneuvers that precisely modifiy the trajectory of
New Horizons to aim the approach.
On January 15, 2015, NASA gave a brief update of the timeline of the approach and departure phases.
[91]
|
January 2015: NASA updates New Horizons timeline.
|
|
On February 12, 2015, NASA released new images of Pluto (taken from January 25 to 31) from the approaching probe.
[92][93] New Horizons was more than 203,000,000 km (126,000,000 mi) away from Pluto when it began taking the photos, which showed Pluto and its largest moon, Charon. The exposure time was too short to see Pluto’s smaller, much fainter moons.
|
January 2015: New Horizons takes images of Pluto and its largest moon, Charon.
|
|
Investigators compiled a series of images of the moons Nix and Hydra taken from January 27 through February 8, 2015, beginning at a range of 201,000,000 km (125,000,000 mi).
[94] Pluto and Charon appear as a single overexposed object at the center. The right side image has been processed to remove the background starfield.
|
January and February 2015: New Horizons takes images of Pluto, Charon, Nix, and Hydra.
|
|
Also in regards to this approach phase during January 2015, on August 21, 2012, the team had announced that they would also spend mission time attempting long-range observations of the Kuiper belt object, temporarily designated
VNH0004, when the object was at a distance from
New Horizons of 75 gigameters (0.50 AU).
[95] The object would be too distant to resolve surface features or take spectroscopy, but it would be able to make observations that cannot be made from Earth, namely a
phase curve and a search for small moons. A second object will be observed in June, and a third in September, after the flyby; the team hopes to observe a dozen such objects through 2018.
[95]
Current status
The latest news and photos from the probe can be viewed at
NASA's Web site.
As of February 28, 2015,
New Horizons was about:
- 1.08 AU (162,000,000 km; 100,000,000 mi) from Pluto
- 31.78 AU (4.754×109 km; 2.954×109 mi) from the Sun
- 32.35 AU (4.839×109 km; 3.007×109 mi) from Earth.
The spacecraft traveled at 14.6 km/s (33,000 mph) or about 3.0
AU per year (relative to the Sun). Radio signals take four and a half hours to travel to the spacecraft from Earth.
[96]
The brightness of the Sun from the spacecraft is
magnitude −19.3.
[97] New Horizons is heading in the direction of the constellation
Sagittarius.
[97]
Future mission timeline
Pluto flyby
New Horizons approaching
Pluto and
Charon (artist concept).
Computer-generated simulation of what the view from the spacecraft might look like during the flyby of
Pluto (right) also showing its moon,
Charon (left)
[citation needed]
On May 5, 2015,
New Horizons ' imaging resolution of Pluto will exceed that of the
Hubble's,
[33] lasting until two weeks after the flyby. From the May date forward on approach the probe should be able to detect any rings or any additional moons (eventually down to 2 km diameter), for avoidance and targeting maneuvers, and observation scheduling.
New Horizons ' best spatial resolution of the small satellites is: 460m/pixel at Nix, 1.1 km/pixel at Hydra, 3.2 km/pixel at Kerberos, and 3.2 km/pixel at Styx. Estimates for the sizes of these bodies (assuming albedo 0.35) are Hydra 60 km, Nix 45 km, Kerberos 13 km, Styx 10 km. This translates to ~55, ~95, ~4, and ~3 pixels in width for Hydra, Nix, Kerberos, and Styx, respectively.
New Horizons is intended to pass within 10,000 km (6,200 mi) of Pluto, with this closest approach date estimated to occur on July 14, 2015 at 11:50 UTC.
New Horizons will have a relative velocity of 13.78 km/s (49,600 km/h; 30,800 mph) at its closest approach, and will come as close as 27,000 km (17,000 mi) to
Charon, although these parameters may be changed during flight. Long-range imaging will include 40 km (25 mi) mapping of Pluto and Charon 3.2
days out. This is half the rotation period of Pluto–Charon and will allow imaging of the side of both bodies that will be facing away from the spacecraft at closest approach. Coverage will repeat twice per day, to search for changes due to snows or
cryovolcanism. Still, due to Pluto's tilt and rotation, a portion of the northern hemisphere will be in shadow at all times. During the flyby, LORRI should be able to obtain select images with resolution as high as 50
m/
px (if closest distance is around 10,000 km), and MVIC should obtain 4-color global dayside maps at 1.6 km resolution. LORRI and MVIC will attempt to overlap their respective coverage areas to form stereo pairs. LEISA will obtain hyperspectral near-infrared maps at 7 km/px globally and 0.6 km/pixel for selected areas.
Meanwhile, Alice will characterize the atmosphere, both by emissions of atmospheric molecules (
airglow), and by dimming of background stars as they pass behind Pluto (
occultation). During and after closest approach, SWAP and PEPSSI will sample the high atmosphere and its effects on the
solar wind. VBSDC will search for dust, inferring meteoroid collision rates and any invisible rings. REX will perform active and passive radio science. Ground stations on Earth will transmit a powerful radio signal as
New Horizons passes behind Pluto's disk, then emerges on the other side. The communications dish will measure the disappearance and reappearance of the
radio occultation signal. The results will resolve Pluto's diameter (by their timing) and atmospheric density and composition (by their weakening and strengthening pattern). (Alice can perform similar occultations, using sunlight instead of radio beacons.) Previous missions had the spacecraft transmit through the atmosphere, to Earth ("downlink"). Low power and extreme distance means
New Horizons will be the first such "uplink" mission. Pluto's mass and mass distribution will be evaluated by their tug on the spacecraft. As the spacecraft speeds up and slows down, the radio signal will experience a
Doppler shift. The Doppler shift will be measured by comparison with the ultrastable oscillator in the communications electronics.
Reflected sunlight from Charon will allow some imaging observations of the nightside. Backlighting by the Sun will highlight any rings or atmospheric hazes. REX will perform radiometry of the nightside.
Initial, highly-
compressed images will be transmitted within days. The science team will select the best images for public release. Uncompressed images will take about nine months
[citation needed] to transmit, depending on
Deep Space Network traffic. It may turn out that fewer months will be needed. The spacecraft link is proving stronger than expected, and it is possible that both downlink channels may be ganged together to nearly double the data rate.
- Primary objectives (required)
- Characterize the global geology and morphology of Pluto and Charon
- Map chemical compositions of Pluto and Charon surfaces
- Characterize the neutral (non-ionized) atmosphere of Pluto and its escape rate
Loss of any of these objectives will constitute a partial failure of the mission.
- Secondary objectives (expected)
- Characterize the time variability of Pluto's surface and atmosphere
- Image select Pluto and Charon areas in stereo
- Map the terminators (day/night border) of Pluto and Charon with high resolution
- Map the chemical compositions of select Pluto and Charon areas with high resolution
- Characterize Pluto's ionosphere (upper layer of the atmosphere), and its interaction with the solar wind
- Search for neutral species such as H2, hydrocarbons, HCN and other nitriles in the atmosphere
- Search for any Charon atmosphere
- Determine bolometric Bond albedos for Pluto and Charon
- Map surface temperatures of Pluto and Charon
- Map any additional surfaces of outer most moons; Nix, Hydra, Kerberos & Styx.
It is expected, but not demanded, that most of these objectives will be met.
- Tertiary objectives (desired)
- Characterize the energetic particle environment at Pluto and Charon
- Refine bulk parameters (radii, masses) and orbits of Pluto and Charon
- Search for additional moons, and any rings
These objectives may be attempted, though they may be skipped in favor of the above objectives. An objective to measure any magnetic field of Pluto was dropped. A
magnetometer instrument could not be implemented within a reasonable mass budget and schedule, and SWAP and PEPSSI could do an indirect job detecting some magnetic field around Pluto.
Kuiper belt object mission
A comparison of Pluto's orbit, the Kuiper belt, and the presumed distance of the Oort cloud compared to the rest of the Solar System
Target background
After passing by Pluto,
New Horizons will continue farther into the
Kuiper belt. The goal is to study one or two other
Kuiper belt objects. Because maneuvering capability is limited, this phase of the mission is contingent on the presence of suitable KBOs close to
New Horizons ' flight path (which ruled out any possibility for a flyby of
Eris, a
trans-Neptunian object comparable in size to Pluto.
[99]). Because the flight path is determined by the Pluto flyby, with only minimal
hydrazine remaining, objects needed to be found within a cone, extending from Pluto, of less than a degree's width, within 55
AU. Past 55
AU, the communications link will become too weak, and the RTG wattage will have decayed significantly enough to hinder observations. Desirable KBOs will be well over 50 km (31 mi) in diameter, neutral in color (to compare with the reddish Pluto), and, if possible, possess a moon. Because the population of KBOs appears quite large, multiple objects may qualify. Mission planners searched for one or more additional Kuiper belt objects (KBOs) of the order of 50–100 km (31–62 mi) in diameter as targets for flybys similar to the spacecraft's Plutonian encounter. The available region, being fairly close to the plane of the Milky
Way and thus difficult to survey for dim objects, is one that was not well-covered by previous KBO search efforts.
Search
In 2011 a dedicated search for suitable KBOs using ground telescopes was started. Large ground telescopes with wide-field cameras, notably the twin 6.5-meter
Magellan Telescopes in Chile, the 8.2-meter
Subaru Observatory in Hawaii, and the
Canada-France-Hawaii Telescope[100][101] were used to search for potential targets. Through the
citizen science project, the public helped to scan telescopic images for possible suitable mission candidates by participating in the
Ice Hunters project.
[102][103][104][105][106] The ground-based search resulted in the discovery of about 143 KBOs of potential interest,
[107] but none of these were close enough to the flight path of
New Horizons.
[100]
Only the
Hubble Space Telescope was deemed likely to find a suitable target in time for a successful KBO mission.
[108] On June 16, 2014, time on Hubble was granted.
[109] Hubble has a much greater ability to find suitable KBOs than ground telescopes. The probability that a target for
New Horizons would be found was estimated beforehand at about 95%.
[110]
Suitable KBOs
On October 15, 2014, it was revealed that Hubble's search had uncovered three potential targets,
[98] [111][112][113] provisionally designated PT1, PT2 and PT3 by the
New Horizons team. All are objects with estimated diameters in the 30–55 km range, too small to be sighted by ground telescopes, at distances from the Sun of 43–44 AU, which would put the encounters in the 2018–2019 period.
[111] The initial estimated probabilities that these objects are reachable within
New Horizons ' fuel budget are 100%, 97% and 7%, respectively.
[111] All are members of the "cold" (low-
inclination, low-
eccentricity)
classical Kuiper belt, and thus very different from Pluto. PT1 (designated
1110113Y on the HST web site
[114]), the most favorably situated object, is magnitude 26.8, 30–45 km in diameter, and would be encountered around January 2019. A course to reach it would require about 35% of
New Horizons ' available
trajectory-adjustment fuel supply. A mission to PT2 or PT3 may be preferable, as they are brighter and therefore probably larger than PT1.
[111]
Possible Neptune trojan objectives
Lagrange points in the Sun-Earth system (not to scale)
Other possible targets were
Neptune trojans. The probe's trajectory to Pluto passed near
Neptune's trailing
Lagrange point ("
L5"), which may host hundreds of bodies in 1:1
resonance. In late 2013,
New Horizons passed within 1.2 AU (180,000,000 km; 110,000,000 mi) of the high-inclination L5 Neptune trojan
2011 HM102,
[101] which was identified shortly before by the
New Horizons KBO Search Survey team while searching for more distant objects for
New Horizons to fly by after its 2015 Pluto encounter. At that range,
2011 HM102 would have been bright enough to be detectable by
New Horizons ' LORRI instrument. The
2011 HM102 flyby came shortly before the Pluto encounter. At that time,
New Horizons may not have had significant downlink bandwidth, and thus free memory, for trojan encounter data.
[115] The
New Horizons team eventually decided that they would not target
2011 HM102 for observations because the preparations for the Pluto approach took precedence.
[116]
Post KBO mission - the heliosphere and science conclusion
Provided it survives that far out,
New Horizons is likely to follow the
Voyager probes in exploring the outer
heliosphere and mapping the heliosheath and heliopause. The heliopause might be reached around year 2047.
[117]
Even though it was launched far faster than any outward probe before it,
New Horizons will never overtake either
Voyager 1 or
Voyager 2, as the most distant human-made object from Earth. Close fly-bys of
Saturn and
Titan gave
Voyager 1 an advantage with its extra
gravity assist. When
New Horizons reaches the distance of
100 AU, it will be travelling at about 13 km/s (29,000 mph), around 4 km/s (8,900 mph) slower than
Voyager 1 at that distance.
[54]
A public-defined mission not proposed by the New Horizons team
In August 2013, it was proposed
[118] to use the radio-tracking data from
New Horizons to put constraints on the position of a hypothesized
trans-Neptunian planetary-sized body.
[119]