Water resources are natural resources of water that are potentially useful for humans, for example as a source of drinking water supply or irrigation water. 97% of the water on Earth is salt water and only three percent is fresh water; slightly over two-thirds of this is frozen in glaciers and polar ice caps. The remaining unfrozen freshwater is found mainly as groundwater, with only a small fraction present above ground or in the air. Natural sources of fresh water include surface water, under river flow, groundwater and frozen water. Non-natural or human-made sources of fresh water can include wastewater that has been treated for reuse options, and desalinated seawater. People use water resources for agricultural, industrial and household activities.
Water resources are under threat from multiple issues. There is water scarcity, water pollution, water conflict and climate change. Fresh water is in principle a renewable resource. However, the world's supply of groundwater is steadily decreasing. Groundwater depletion (or overdrafting) is occurring for example in Asia, South America and North America.
Natural sources of fresh water
Distribution of Freshwater Resources by Type
Natural sources of fresh water include surface water, under river flow, groundwater and frozen water.
Surface water

Surface water is water in a river, lake or fresh water wetland. Surface water is naturally replenished by precipitation and naturally lost through discharge to the oceans, evaporation, evapotranspiration and groundwater recharge. The only natural input to any surface water system is precipitation within its watershed. The total quantity of water in that system at any given time is also dependent on many other factors. These factors include storage capacity in lakes, wetlands and artificial reservoirs, the permeability of the soil beneath these storage bodies, the runoff characteristics of the land in the watershed, the timing of the precipitation and local evaporation rates. All of these factors also affect the proportions of water loss.
Humans often increase storage capacity by constructing reservoirs and decrease it by draining wetlands. Humans often increase runoff quantities and velocities by paving areas and channelizing the stream flow.
Natural surface water can be augmented by importing surface water from another watershed through a canal or pipeline.
Brazil is estimated to have the largest supply of fresh water in the world, followed by Russia and Canada.
-
Panorama of a natural wetland (Sinclair Wetlands, New Zealand)
Water from glaciers
Glacier runoff is considered to be surface water. The Himalayas, which are often called "The Roof of the World", contain some of the most extensive and rough high altitude areas on Earth as well as the greatest area of glaciers and permafrost outside of the poles. Ten of Asia's largest rivers flow from there, and more than a billion people's livelihoods depend on them. To complicate matters, temperatures there are rising more rapidly than the global average. In Nepal, the temperature has risen by 0.6 degrees Celsius over the last decade, whereas globally, the Earth has warmed approximately 0.7 degrees Celsius over the last hundred years.
Groundwater

Groundwater is the water present beneath Earth's surface in rock and soil pore spaces and in the fractures of rock formations. About 30 percent of all readily available freshwater in the world is groundwater. A unit of rock or an unconsolidated deposit is called an aquifer when it can yield a usable quantity of water. The depth at which soil pore spaces or fractures and voids in rock become completely saturated with water is called the water table. Groundwater is recharged from the surface; it may discharge from the surface naturally at springs and seeps, and can form oases or wetlands. Groundwater is also often withdrawn for agricultural, municipal, and industrial use by constructing and operating extraction wells. The study of the distribution and movement of groundwater is hydrogeology, also called groundwater hydrology.
Typically, groundwater is thought of as water flowing through shallow aquifers, but, in the technical sense, it can also contain soil moisture, permafrost (frozen soil), immobile water in very low permeability bedrock, and deep geothermal or oil formation water. Groundwater is hypothesized to provide lubrication that can possibly influence the movement of faults. It is likely that much of Earth's subsurface contains some water, which may be mixed with other fluids in some instances.Under river flow
Throughout the course of a river, the total volume of water transported downstream will often be a combination of the visible free water flow together with a substantial contribution flowing through rocks and sediments that underlie the river and its floodplain called the hyporheic zone. For many rivers in large valleys, this unseen component of flow may greatly exceed the visible flow. The hyporheic zone often forms a dynamic interface between surface water and groundwater from aquifers, exchanging flow between rivers and aquifers that may be fully charged or depleted. This is especially significant in karst areas where pot-holes and underground rivers are common.
Artificial sources of usable water
There are several artificial sources of fresh water. One is treated wastewater (reclaimed water). Another is atmospheric water generators.[8][9][10] Desalinated seawater is another important source. It is important to consider the economic and environmental side effects of these technologies.
Wastewater reuse
Water reclamation is the process of converting municipal wastewater or sewage and industrial wastewater into water that can be reused for a variety of purposes . It is also called wastewater reuse, water reuse or water recycling. There are many types of reuse. It is possible to reuse water in this way in cities or for irrigation in agriculture. Other types of reuse are environmental reuse, industrial reuse, and reuse for drinking water, whether planned or not. Reuse may include irrigation of gardens and agricultural fields or replenishing surface water and groundwater. This latter is also known as groundwater recharge. Reused water also serve various needs in residences such as toilet flushing, businesses, and industry. It is possible to treat wastewater to reach drinking water standards. Injecting reclaimed water into the water supply distribution system is known as direct potable reuse. Drinking reclaimed water is not typical. Reusing treated municipal wastewater for irrigation is a long-established practice. This is especially so in arid countries. Reusing wastewater as part of sustainable water management allows water to remain an alternative water source for human activities. This can reduce scarcity. It also eases pressures on groundwater and other natural water bodies.
There are several technologies used to treat wastewater for reuse. A combination of these technologies can meet strict treatment standards and make sure that the processed water is hygienically safe, meaning free from pathogens. The following are some of the typical technologies: Ozonation, ultrafiltration, aerobic treatment (membrane bioreactor), forward osmosis, reverse osmosis, and advanced oxidation, or activated carbon. Some water-demanding activities do not require high grade water. In this case, wastewater can be reused with little or no treatment.Desalinated water
Desalination is a process that removes mineral components from saline water. More generally, desalination is the removal of salts and minerals from a substance. One example is soil desalination. This is important for agriculture. It is possible to desalinate saltwater, especially sea water, to produce water for human consumption or irrigation. The by-product of the desalination process is brine. Many seagoing ships and submarines use desalination. Modern interest in desalination mostly focuses on cost-effective provision of fresh water for human use. Along with recycled wastewater, it is one of the few water resources independent of rainfall.
Due to its energy consumption, desalinating sea water is generally more costly than fresh water from surface water or groundwater, water recycling and water conservation; however, these alternatives are not always available and depletion of reserves is a critical problem worldwide. Desalination processes are using either thermal methods (in the case of distillation) or membrane-based methods (e.g. in the case of reverse osmosis) energy types.Research into other options
Air-capture over oceans

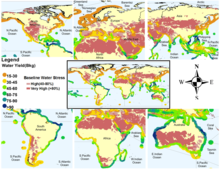
Researchers proposed "significantly increasing freshwater through the capture of humid air over oceans" to address present and, especially, future water scarcity/insecurity.
Atmospheric water generators on land
A potentials-assessment study proposed hypothetical portable solar-powered atmospheric water harvesting devices which are under development, along with design criteria, finding they could help a billion people to access safe drinking water, albeit such off-the-grid generation may sometimes "undermine efforts to develop permanent piped infrastructure" among other problems.
Water uses

The total quantity of water available at any given time is an important consideration. Some human water users have an intermittent need for water. For example, many farms require large quantities of water in the spring, and no water at all in the winter. Other users have a continuous need for water, such as a power plant that requires water for cooling. Over the long term the average rate of precipitation within a watershed is the upper bound for average consumption of natural surface water from that watershed.
Agriculture and other irrigation
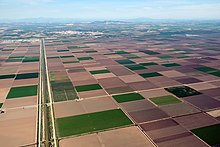
Irrigation (also referred to as watering of plants) is the practice of applying controlled amounts of water to land to help grow crops, landscape plants, and lawns. Irrigation has been a key aspect of agriculture for over 5,000 years and has been developed by many cultures around the world. Irrigation helps to grow crops, maintain landscapes, and revegetate disturbed soils in dry areas and during times of below-average rainfall. In addition to these uses, irrigation is also employed to protect crops from frost, suppress weed growth in grain fields, and prevent soil consolidation. It is also used to cool livestock, reduce dust, dispose of sewage, and support mining operations. Drainage, which involves the removal of surface and sub-surface water from a given location, is often studied in conjunction with irrigation.
There are several methods of irrigation that differ in how water is supplied to plants. Surface irrigation, also known as gravity irrigation, is the oldest form of irrigation and has been in use for thousands of years. In sprinkler irrigation, water is piped to one or more central locations within the field and distributed by overhead high-pressure water devices. Micro-irrigation is a system that distributes water under low pressure through a piped network and applies it as a small discharge to each plant. Micro-irrigation uses less pressure and water flow than sprinkler irrigation. Drip irrigation delivers water directly to the root zone of plants. Subirrigation has been used in field crops in areas with high water tables for many years. It involves artificially raising the water table to moisten the soil below the root zone of plants.
Irrigation water can come from groundwater (extracted from springs or by using wells), from surface water (withdrawn from rivers, lakes or reservoirs) or from non-conventional sources like treated wastewater, desalinated water, drainage water, or fog collection. Irrigation can be supplementary to rainfall, which is common in many parts of the world as rainfed agriculture, or it can be full irrigation, where crops rarely rely on any contribution from rainfall. Full irrigation is less common and only occurs in arid landscapes with very low rainfall or when crops are grown in semi-arid areas outside of rainy seasons.Industries
It is estimated that 22% of worldwide water is used in industry. Major industrial users include hydroelectric dams, thermoelectric power plants, which use water for cooling, ore and oil refineries, which use water in chemical processes, and manufacturing plants, which use water as a solvent. Water withdrawal can be very high for certain industries, but consumption is generally much lower than that of agriculture.
Water is used in renewable power generation. Hydroelectric power derives energy from the force of water flowing downhill, driving a turbine connected to a generator. This hydroelectricity is a low-cost, non-polluting, renewable energy source. Significantly, hydroelectric power can also be used for load following unlike most renewable energy sources which are intermittent. Ultimately, the energy in a hydroelectric power plant is supplied by the sun. Heat from the sun evaporates water, which condenses as rain in higher altitudes and flows downhill. Pumped-storage hydroelectric plants also exist, which use grid electricity to pump water uphill when demand is low, and use the stored water to produce electricity when demand is high.
Thermoelectric power plants using cooling towers have high consumption, nearly equal to their withdrawal, as most of the withdrawn water is evaporated as part of the cooling process. The withdrawal, however, is lower than in once-through cooling systems.
Water is also used in many large scale industrial processes, such as thermoelectric power production, oil refining, fertilizer production and other chemical plant use, and natural gas extraction from shale rock. Discharge of untreated water from industrial uses is pollution. Pollution includes discharged solutes and increased water temperature (thermal pollution).
Drinking water and domestic use (households)

It is estimated that 8% of worldwide water use is for domestic purposes. These include drinking water, bathing, cooking, toilet flushing, cleaning, laundry and gardening. Basic domestic water requirements have been estimated by Peter Gleick at around 50 liters per person per day, excluding water for gardens.
Drinking water is water that is of sufficiently high quality so that it can be consumed or used without risk of immediate or long term harm. Such water is commonly called potable water. In most developed countries, the water supplied to domestic, commerce and industry is all of drinking water standard even though only a very small proportion is actually consumed or used in food preparation.
844 million people still lacked even a basic drinking water service in 2017. Of those, 159 million people worldwide drink water directly from surface water sources, such as lakes and streams. One in eight people in the world do not have access to safe water.
Challenges and threats
Water scarcity
Water pollution
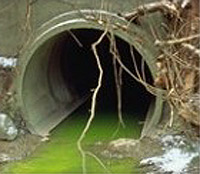
Water conflict
Climate change
Groundwater overdrafting
The world's supply of groundwater is steadily decreasing. Groundwater depletion (or overdrafting) is occurring for example in Asia, South America and North America. It is still unclear how much natural renewal balances this usage, and whether ecosystems are threatened.
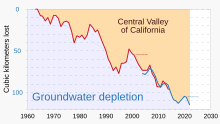
Water resource management

Water resource management is the activity of planning, developing, distributing and managing the optimum use of water resources. It is an aspect of water cycle management. The field of water resources management will have to continue to adapt to the current and future issues facing the allocation of water. With the growing uncertainties of global climate change and the long-term impacts of past management actions, this decision-making will be even more difficult. It is likely that ongoing climate change will lead to situations that have not been encountered. As a result, alternative management strategies, including participatory approaches and adaptive capacity are increasingly being used to strengthen water decision-making.
Ideally, water resource management planning has regard to all the competing demands for water and seeks to allocate water on an equitable basis to satisfy all uses and demands. As with other resource management, this is rarely possible in practice so decision-makers must prioritise issues of sustainability, equity and factor optimisation (in that order!) to achieve acceptable outcomes. One of the biggest concerns for water-based resources in the future is the sustainability of the current and future water resource allocation.
Sustainable Development Goal 6 has a target related to water resources management: "Target 6.5: By 2030, implement integrated water resources management at all levels, including through transboundary cooperation as appropriate."
Sustainable water management
At present, only about 0.08 percent of all the world's fresh water is accessible. And there is ever-increasing demand for drinking, manufacturing, leisure and agriculture. Due to the small percentage of water available, optimizing the fresh water we have left from natural resources has been a growing challenge around the world.
Much effort in water resource management is directed at optimizing the use of water and in minimizing the environmental impact of water use on the natural environment. The observation of water as an integral part of the ecosystem is based on integrated water resources management, based on the 1992 Dublin Principles (see below).
Sustainable water management requires a holistic approach based on the principles of Integrated Water Resource Management, originally articulated in 1992 at the Dublin (January) and Rio (July) conferences. The four Dublin Principles, promulgated in the Dublin Statement are:
- Fresh water is a finite and vulnerable resource, essential to sustain life, development and the environment;
- Water development and management should be based on a participatory approach, involving users, planners and policy-makers at all levels;
- Women play a central part in the provision, management and safeguarding of water;
- Water has an economic value in all its competing uses and should be recognized as an economic good.
Implementation of these principles has guided reform of national water management law around the world since 1992.
Further challenges to sustainable and equitable water resources management include the fact that many water bodies are shared across boundaries which may be international (see water conflict) or intra-national (see Murray-Darling basin).
Integrated water resources management
Integrated water resources management (IWRM) has been defined by the Global Water Partnership (GWP) as "a process which promotes the coordinated development and management of water, land and related resources, in order to maximize the resultant economic and social welfare in an equitable manner without compromising the sustainability of vital ecosystems".
Some scholars say that IWRM is complementary to water security because water security is a goal or destination, whilst IWRM is the process necessary to achieve that goal.
IWRM is a paradigm that emerged at international conferences in the late 1900s and early 2000s, although participatory water management institutions have existed for centuries. Discussions on a holistic way of managing water resources began already in the 1950s leading up to the 1977 United Nations Water Conference. The development of IWRM was particularly recommended in the final statement of the ministers at the International Conference on Water and the Environment in 1992, known as the Dublin Statement. This concept aims to promote changes in practices which are considered fundamental to improved water resource management. IWRM was a topic of the second World Water Forum, which was attended by a more varied group of stakeholders than the preceding conferences and contributed to the creation of the GWP.
In the International Water Association definition, IWRM rests upon three principles that together act as the overall framework:
- Social equity: ensuring equal access for all users (particularly marginalized and poorer user groups) to an adequate quantity and quality of water necessary to sustain human well-being.
- Economic efficiency: bringing the greatest benefit to the greatest number of users possible with the available financial and water resources.
- Ecological sustainability: requiring that aquatic ecosystems are acknowledged as users and that adequate allocation is made to sustain their natural functioning.
In 2002, the development of IWRM was discussed at the World Summit on Sustainable Development held in Johannesburg, which aimed to encourage the implementation of IWRM at a global level.[58] The third World Water Forum recommended IWRM and discussed information sharing, stakeholder participation, and gender and class dynamics.
Operationally, IWRM approaches involve applying knowledge from various disciplines as well as the insights from diverse stakeholders to devise and implement efficient, equitable and sustainable solutions to water and development problems. As such, IWRM is a comprehensive, participatory planning and implementation tool for managing and developing water resources in a way that balances social and economic needs, and that ensures the protection of ecosystems for future generations. In addition, in light of contributing the achievement of Sustainable Development goals (SDGs), IWRM has been evolving into more sustainable approach as it considers the Nexus approach, which is a cross-sectoral water resource management. The Nexus approach is based on the recognition that "water, energy and food are closely linked through global and local water, carbon and energy cycles or chains."
An IWRM approach aims at avoiding a fragmented approach of water resources management by considering the following aspects: Enabling environment, roles of Institutions, management Instruments. Some of the cross-cutting conditions that are also important to consider when implementing IWRM are: Political will and commitment, capacity development, adequate investment, financial stability and sustainable cost recovery, monitoring and evaluation. There is not one correct administrative model. The art of IWRM lies in selecting, adjusting and applying the right mix of these tools for a given situation. IWRM practices depend on context; at the operational level, the challenge is to translate the agreed principles into concrete action.
Managing water in urban settings
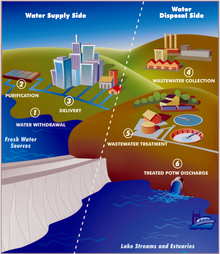
Integrated urban water management (IUWM) is the practice of managing freshwater, wastewater, and storm water as components of a basin-wide management plan. It builds on existing water supply and sanitation considerations within an urban settlement by incorporating urban water management within the scope of the entire river basin. IUWM is commonly seen as a strategy for achieving the goals of Water Sensitive Urban Design. IUWM seeks to change the impact of urban development on the natural water cycle, based on the premise that by managing the urban water cycle as a whole; a more efficient use of resources can be achieved providing not only economic benefits but also improved social and environmental outcomes. One approach is to establish an inner, urban, water cycle loop through the implementation of reuse strategies. Developing this urban water cycle loop requires an understanding both of the natural, pre-development, water balance and the post-development water balance. Accounting for flows in the pre- and post-development systems is an important step toward limiting urban impacts on the natural water cycle.
IUWM within an urban water system can also be conducted by performance assessment of any new intervention strategies by developing a holistic approach which encompasses various system elements and criteria including sustainability type ones in which integration of water system components including water supply, waste water and storm water subsystems would be advantageous. Simulation of metabolism type flows in urban water system can also be useful for analysing processes in urban water cycle of IUWM.