From Wikipedia, the free encyclopedia
Nile | |
River | |
Countries | Ethiopia, Sudan, Egypt, Uganda, Congo-Kinshasa, Kenya, Tanzania, Rwanda, Burundi, South Sudan, Eritrea |
---|---|
Cities | Jinja, Juba, Khartoum, Cairo |
Primary source | White Nile |
- elevation | 2,700 m (8,858 ft) |
- coordinates | 02°16′56″S 029°19′53″E / 2.28222°S 29.33139°E |
Secondary source | Blue Nile |
- location | Lake Tana, Ethiopia |
- coordinates | 12°02′09″N 037°15′53″E / 12.03583°N 37.26472°E |
Source confluence | near Khartoum |
Mouth | |
- location | Mediterranean Sea, Egypt |
- elevation | 0 m (0 ft) |
- coordinates | 30°10′N 031°06′E / 30.167°N 31.100°E [1] |
Length | 6,853 km (4,258 mi) |
Width | 2.8 km (2 mi) |
Basin | 3,400,000 km2 (1,312,747 sq mi) |
Discharge | |
- average | 2,830 m3/s (99,941 cu ft/s) |
The Nile (Arabic: النيل, Eg. en-Nīl, Std. an-Nīl; Coptic: ⲫⲓⲁⲣⲱ, P(h)iaro; Ancient Egyptian: Ḥ'pī and Iteru) is a major north-flowing river in northeastern Africa, generally regarded as the longest river in the world.[2] It is 6,853 km (4,258 miles) long. The Nile is an "international" river as its water resources are shared by eleven countries, namely, Tanzania, Uganda, Rwanda, Burundi, Congo-Kinshasa, Kenya, Ethiopia, Eritrea, South Sudan, Sudan and Egypt.[3] In particular, the Nile is the primary water source of Egypt and Sudan.[4]
The Nile has two major tributaries, the White Nile and Blue Nile. The White Nile is considered to be the headwaters and primary stream of the Nile itself. The Blue Nile, however, is the source of most of the water and fertile soil. The White Nile is longer and rises in the Great Lakes region of central Africa, with the most distant source still undetermined but located in either Rwanda or Burundi. It flows north through Tanzania, Lake Victoria, Uganda and South Sudan. The Blue Nile (Amharic: ዓባይ?, ʿĀbay[5][6]) begins at Lake Tana in Ethiopia[7] and flows into Sudan from the southeast. The two rivers meet near the Sudanese capital of Khartoum.
The northern section of the river flows north almost entirely through the Sudanese desert to Egypt, then ends in a large delta and empties into the Mediterranean Sea. Egyptian civilization and Sudanese kingdoms have depended on the river since ancient times. Most of the population and cities of Egypt lie along those parts of the Nile valley north of Aswan, and nearly all the cultural and historical sites of Ancient Egypt are found along riverbanks.
In the ancient Egyptian language, the Nile is called Ḥ'pī or Iteru, meaning "river", represented by the hieroglyphs shown on the left (literally itrw, and 'waters' determinative).[8] In Coptic, the words piaro (Sahidic) or phiaro (Bohairic) meaning "the river" (lit. p(h).iar-o "the.canal-great") come from the same ancient name.
The English name Nile and the Arabic names en-Nîl and an-Nîl both derive from the Latin Nilus and the Ancient Greek Νεῖλος.[9][10] Beyond that, however, the etymology is disputed.[10][11] One possible etymology derives it from a Semitic Nahal, meaning "river".[12] The standard English names "White Nile" and "Blue Nile" to refer to the river's headwaters derive from Arabic names formerly applied only to the Sudanese stretches which meet at Khartoum.[10]
Course

Above Khartoum the Nile is also known as the White Nile, a term also used in a limited sense to describe the section between Lake No and Khartoum. At Khartoum the river is joined by the Blue Nile. The White Nile starts in equatorial East Africa, and the Blue Nile begins in Ethiopia. Both branches are on the western flanks of the East African Rift.
The drainage basin of the Nile covers 3,254,555 square kilometers (1,256,591 sq mi), about 10% of the area of Africa.[14] The Nile basin is complex, and because of this, the discharge at any given point along the mainstem depends on many factors including weather, diversions, evaporation and evapotranspiration, and groundwater flow.
Sources
The source of the Nile is sometimes considered to be Lake Victoria, but the lake has feeder rivers of considerable size. The Kagera River, which flows into Lake Victoria near the Tanzanian town of Bukoba, is the longest feeder, although sources do not agree on which is the longest tributary of the Kagera and hence the most distant source of the Nile itself.[15] It is either the Ruvyironza, which emerges in Bururi Province, Burundi,[16] or the Nyabarongo, which flows from Nyungwe Forest in Rwanda.[17] The two feeder rivers meet near Rusumo Falls on the Rwanda-Tanzania border.In 2010, an exploration party[18] went to a place described as the source of the Rukarara tributary,[19] and by hacking a path up steep jungle-choked mountain slopes in the Nyungwe forest found (in the dry season) an appreciable incoming surface flow for many kilometres upstream, and found a new source, giving the Nile a length of 6,758 km (4,199 mi)
Gish Abay is reportedly the place where the "holy water" of the first drops of the Nile develop.[20]
Lost headwaters
Formerly Lake Tanganyika drained northwards along the African Rift Valley into the White Nile, making the Nile about 1,400 kilometers (870 mi) longer, until it was blocked in Miocene times by the bulk of the Virunga Volcanoes.In Uganda
The Nile leaves Lake Nyanza (Victoria) at Ripon Falls near Jinja, Uganda, as the Victoria Nile. It flows north for some 130 kilometers (81 mi), to Lake Kyoga. The last part of the approximately 200 kilometers (120 mi) river section starts from the western shores of the lake and flows at first to the west until just south of Masindi Port, where the river turns north, then makes a great half circle to the east and north until Karuma Falls. For the remaining part it flows merely westernly through the Murchison Falls until it reaches the very northern shores of Lake Albert where it forms a significant river delta. The lake it self is on the border of DR Congo, but the Nile is not a border river at this point. After leaving Lake Albert, the river continues north through Uganda and is known as the Albert Nile.In South Sudan
The river flows into South Sudan just south of Nimule, where it is known as the Bahr al Jabal ("Mountain River"[21]). Just south of the town it has the confluence with the Achwa River. The Bahr al Ghazal, itself 716 kilometers (445 mi) long, joins the Bahr al Jabal at a small lagoon called Lake No, after which the Nile becomes known as the Bahr al Abyad, or the White Nile, from the whitish clay suspended in its waters. When the Nile floods it leaves a rich silty deposit which fertilizes the soil. The Nile no longer floods in Egypt since the completion of the Aswan Dam in 1970. An anabranch river, the Bahr el Zeraf, flows out of the Nile's Bahr al Jabal section and rejoins the White Nile.The flow rate of the Bahr al Jabal at Mongalla, South Sudan is almost constant throughout the year and averages 1,048 m3/s (37,000 cu ft/s). After Mongalla, the Bahr Al Jabal enters the enormous swamps of the Sudd region of South Sudan. More than half of the Nile's water is lost in this swamp to evaporation and transpiration. The average flow rate of the White Nile at the tails of the swamps is about 510 m3/s (18,000 cu ft/s). From here it soon meets with the Sobat River at Malakal. On an annual basis, the White Nile upstream of Malakal contributes about fifteen percent of the total outflow of the Nile River.[22]
The average flow of the White Nile at Malakal, just below the Sobat River, is 924 m3/s (32,600 cu ft/s); the peak flow is approximately 1,218 m3/s (43,000 cu ft/s) in October and minimum flow is about 609 m3/s (21,500 cu ft/s) in April. This fluctuation is due the substantial variation in the flow of the Sobat, which has a minimum flow of about 99 m3/s (3,500 cu ft/s) in March and a peak flow of over 680 m3/s (24,000 cu ft/s) in October.[23] During the dry season (January to June) the White Nile contributes between 70 percent and 90 percent of the total discharge from the Nile.
In Sudan
Below Renk the White Nile enters Sudan, it flows north to Khartoum and meets the Blue Nile.The course of the Nile in Sudan is distinctive. It flows over six groups of cataracts, from the first at Aswan to the sixth at Sabaloka (just north of Khartoum) and then turns to flow southward before again returning to flow north. One name for this is the "Great Bend".[citation needed]
In the north of Sudan the river enters Lake Nasser (known in Sudan as Lake Nubia), the larger part of which is in Egypt.
In Egypt
Below the Aswan High Dam, at the northern limit of Lake Nasser, the Nile resumes its historic course.North of Cairo, the Nile splits into two branches (or distributaries) that feed the Mediterranean: the Rosetta Branch to the west and the Damietta to the east, forming the Nile Delta.
Tributaries
Atbara River
Below the confluence with the Blue Nile the only major tributary is the Atbara River, roughly halfway to the sea, which originates in Ethiopia north of Lake Tana, and is around 800 kilometers (500 mi) long. The Atbara flows only while there is rain in Ethiopia and dries very rapidly. During the dry period of January to June, it typically dries up. It joins the Nile approximately 300 kilometers (200 mi) north of Khartoum.Blue Nile

The Blue Nile (Ge'ez ጥቁር ዓባይ Ṭiqūr ʿĀbbāy (Black Abay) to Ethiopians; Arabic: النيل الأزرق; transliterated: an-Nīl al-Azraq) springs from Lake Tana in the Ethiopian Highlands. The Blue Nile flows about 1,400 kilometres to Khartoum, where the Blue Nile and White Nile join to form the Nile. Ninety percent of the water and ninety-six percent of the transported sediment carried by the Nile[25] originates in Ethiopia, with fifty-nine percent of the water from the Blue Nile (the rest being from the Tekezé, Atbarah, Sobat, and small tributaries). The erosion and transportation of silt only occurs during the Ethiopian rainy season in the summer, however, when rainfall is especially high on the Ethiopian Plateau; the rest of the year, the great rivers draining Ethiopia into the Nile (Sobat, Blue Nile, Tekezé, and Atbarah) have a weaker flow.
The flow of the Blue Nile varies considerably over its yearly cycle and is the main contribution to the large natural variation of the Nile flow. During the dry season the natural discharge of the Blue Nile can be as low as 113 m3/s (4,000 cu ft/s), although upstream dams regulate the flow of the river. During the wet season the peak flow of the Blue Nile often exceeds 5,663 m3/s (200,000 cu ft/s) in late August (a difference of a factor of 50).
Before the placement of dams on the river the yearly discharge varied by a factor of 15 at Aswan. Peak flows of over 8,212 m3/s (290,000 cu ft/s) occurred during late August and early September, and minimum flows of about 552 m3/s (19,500 cu ft/s) occurred during late April and early May.
Bahr el Ghazal and Sobat River
The Bahr al Ghazal and the Sobat River are the two most important tributaries of the White Nile in terms of discharge.The Bahr al Ghazal's drainage basin is the largest of any of the Nile's sub-basins, measuring 520,000 square kilometers (200,000 sq mi) in size, but it contributes a relatively small amount of water, about 2 m3/s (71 cu ft/s) annually, due to tremendous volumes of water being lost in the Sudd wetlands.
The Sobat River, which joins the Nile a short distance below Lake No, drains about half as much land, 225,000 km2 (86,900 sq mi), but contributes 412 cubic meters per second (14,500 cu ft/s) annually to the Nile.[26] When in flood the Sobat carries a large amount of sediment, adding greatly to the White Nile's color.[27]
Yellow Nile
The Yellow Nile is a former tributary that connected the Ouaddaï Highlands of eastern Chad to the Nile River Valley c. 8000 to c. 1000 BC.[28] Its remains are known as the Wadi Howar. The wadi passes through Gharb Darfur near the northern border with Chad and meets up with the Nile near the southern point of the Great Bend.History
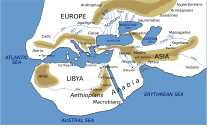
The Nile (iteru in Ancient Egyptian) has been the lifeline of civilization in Egypt since the Stone Age, with most of the population and all of the cities of Egypt resting along those parts of the Nile valley lying north of Aswan. Climate change at the end of the most recent ice age led to the formation of the Sahara desert, possibly as long ago as 3400 BC.
Eonile
The present Nile is at least the fifth river that has flowed north from the Ethiopian Highlands. Satellite imagery was used to identify dry watercourses in the desert to the west of the Nile. An Eonile canyon, now filled by surface drift, represents an ancestral Nile called the Eonile that flowed during the later Miocene (23–5.3 million years before present). The Eonile transported clastic sediments to the Mediterranean; several natural gas fields have been discovered within these sediments.During the late-Miocene Messinian salinity crisis, when the Mediterranean Sea was a closed basin and evaporated to the point of being empty or nearly so, the Nile cut its course down to the new base level until it was several hundred metres below world ocean level at Aswan and 2,400 m (7,900 ft) below Cairo.[29] This created a very long and deep canyon which was filled with sediment when the Mediterranean was recreated. At some point the sediments raised the riverbed sufficiently for the river to overflow westward into a depression to create Lake Moeris.
Lake Tanganyika drained northwards into the Nile until the Virunga Volcanoes blocked its course in Rwanda. The Nile was much longer at that time, with its furthest headwaters in northern Zambia.
Integrated Nile
There are two theories about the age of the integrated Nile. One is that the integrated drainage of the Nile is of young age, and that the Nile basin was formerly broken into series of separate basins, only the most northerly of which fed a river following the present course of the Nile in Egypt and Sudan. Said postulated that Egypt itself supplied most of the waters of the Nile during the early part of its history.[30]The other theory is that the drainage from Ethiopia via rivers equivalent to the Blue Nile and the Atbara and Takazze flowed to the Mediterranean via the Egyptian Nile since well back into Tertiary times.[31]
Salama suggested that during the Paleogene and Neogene Periods (66 million to 2.588 million years ago) a series of separate closed continental basins each occupied one of the major parts of the Sudanese Rift System: Mellut rift, White Nile rift, Blue Nile rift, Atbara rift and Sag El Naam rift.[32] The Mellut Rift Basin is nearly 12 kilometers (7.5 mi) deep at its central part. This rift is possibly still active, with reported tectonic activity in its northern and southern boundaries. The Sudd swamps which form the central part of the basin may still be subsiding. The White Nile Rift System, although shallower than the Bahr el Arab rift, is about 9 kilometers (5.6 mi) deep. Geophysical exploration of the Blue Nile Rift System estimated the depth of the sediments to be 5–9 kilometers (3.1–5.6 mi). These basins were not interconnected until their subsidence ceased, and the rate of sediment deposition was enough to fill and connect them. The Egyptian Nile connected to the Sudanese Nile, which captures the Ethiopian and Equatorial headwaters during the current stages of tectonic activity in the Eastern, Central and Sudanese Rift Systems.[33] The connection of the different Niles occurred during cyclic wet periods. The River Atbara overflowed its closed basin during the wet periods that occurred about 100,000 to 120,000 years ago. The Blue Nile connected to the main Nile during the 70,000–80,000 years B.P. wet period. The White Nile system in Bahr El Arab and White Nile Rifts remained a closed lake until the connection of the Victoria Nile to the main system some 12,500 years ago.
Role in the founding of Egyptian civilization
The Greek historian Herodotus wrote that "Egypt was the gift of the Nile". An unending source of sustenance, it provided a crucial role in the development of Egyptian civilization. Silt deposits from the Nile made the surrounding land fertile because the river overflowed its banks annually. The Ancient Egyptians cultivated and traded wheat, flax, papyrus and other crops around the Nile. Wheat was a crucial crop in the famine-plagued Middle East. This trading system secured Egypt's diplomatic relationships with other countries, and contributed to economic stability. Far-reaching trade has been carried on along the Nile since ancient times. The Ishango bone is probably an early tally stick. It has been suggested that this shows prime numbers and multiplication, but this is disputed. In the book How Mathematics Happened: The First 50,000 Years, Peter Rudman argues that the development of the concept of prime numbers could only have come about after the concept of division, which he dates to after 10,000 BC, with prime numbers probably not being understood until about 500 BC. He also writes that "no attempt has been made to explain why a tally of something should exhibit multiples of two, prime numbers between 10 and 20, and some numbers that are almost multiples of 10."[34] It was discovered along the headwaters of the Nile (near Lake Edward, in northeastern Congo) and was carbon-dated to 20,000 BC.
Water buffalo were introduced from Asia, and Assyrians introduced camels in the 7th century BC. These animals were killed for meat, and were domesticated and used for ploughing—or in the camels' case, carriage. Water was vital to both people and livestock. The Nile was also a convenient and efficient means of transportation for people and goods. The Nile was an important part of ancient Egyptian spiritual life. Hapy was the god of the annual floods, and both he and the pharaoh were thought to control the flooding. The Nile was considered to be a causeway from life to death and the afterlife. The east was thought of as a place of birth and growth, and the west was considered the place of death, as the god Ra, the Sun, underwent birth, death, and resurrection each day as he crossed the sky. Thus, all tombs were west of the Nile, because the Egyptians believed that in order to enter the afterlife, they had to be buried on the side that symbolized death.
As the Nile was such an important factor in Egyptian life, the ancient calendar was even based on the 3 cycles of the Nile. These seasons, each consisting of four months of thirty days each, were called Akhet, Peret, and Shemu. Akhet, which means inundation, was the time of the year when the Nile flooded, leaving several layers of fertile soil behind, aiding in agricultural growth.[35]
Peret was the growing season, and Shemu, the last season, was the harvest season when there were no rains.[35]
Search for the source of the Nile

John Hanning Speke c. 1863. Speke was the Victorian explorer who first reached Lake Victoria in 1858, returning to establish it as the source of the Nile by 1862.[10]

Henry Morton Stanley in 1872. Stanley circumnavigated the lake and confirmed Speke's observations in 1875.[10]
Owing to their failure to penetrate the sudd wetlands of South Sudan, the upper reaches of the Nile remained largely unknown to the ancient Greeks and Romans. Various expeditions failed to determine the river's source. Agatharcides records that in the time of Ptolemy II Philadelphus, a military expedition had penetrated far enough along the course of the Blue Nile to determine that the summer floods were caused by heavy seasonal rainstorms in the Ethiopian Highlands, but no European of antiquity is known to have reached Lake Tana.
The Tabula Rogeriana depicted the source as three lakes in 1154.
Europeans began to learn about the origins of the Nile in the 15th and 16th centuries, when travelers to Ethiopia visited Lake Tana and the source of the Blue Nile in the mountains south of the lake. Although James Bruce claimed to be the first European to have visited the headwaters,[36] modern writers give the credit to the Jesuit Pedro Páez. Páez's account of the source of the Nile[37] is a long and vivid account of Ethiopia. It was published in full only in the early 20th century, although it was featured in works of Páez's contemporaries, including Baltazar Téllez,[38] Athanasius Kircher[39] and by Johann Michael Vansleb.[40]
Europeans had been resident in Ethiopia since the late 15th century, and one of them may have visited the headwaters even earlier without leaving a written trace. The Portuguese João Bermudes published the first description of the Tis Issat Falls in his 1565 memoirs, compared them to the Nile Falls alluded to in Cicero's De Republica.[41] Jerónimo Lobo describes the source of the Blue Nile, visiting shortly after Pedro Páez. Telles also used his account.
The White Nile was even less understood. The ancients mistakenly believed that the Niger River represented the upper reaches of the White Nile. For example, Pliny the Elder wrote that the Nile had its origins "in a mountain of lower Mauretania", flowed above ground for "many days" distance, then went underground, reappeared as a large lake in the territories of the Masaesyli, then sank again below the desert to flow underground "for a distance of 20 days' journey till it reaches the nearest Ethiopians."[42] A merchant named Diogenes reported that the Nile's water attracted game such as buffalo.

A map of the Nile c. 1911, a time when its entire primary course ran through British occupations, condominiums, colonies, and protectorates.[10]
Lake Victoria was first sighted by Europeans in 1858 when the British explorer John Hanning Speke reached its southern shore while traveling with Richard Francis Burton to explore central Africa and locate the great lakes. Believing he had found the source of the Nile on seeing this "vast expanse of open water" for the first time, Speke named the lake after the then Queen of the United Kingdom. Burton, recovering from illness and resting further south on the shores of Lake Tanganyika, was outraged that Speke claimed to have proved his discovery to be the true source of the Nile when Burton regarded this as still unsettled. A very public quarrel ensued, which sparked a great deal of intense debate within the scientific community and interest by other explorers keen to either confirm or refute Speke's discovery. British explorer and missionary David Livingstone pushed too far west and entered the Congo River system instead. It was ultimately Welsh-American explorer Henry Morton Stanley who confirmed Speke's discovery, circumnavigating Lake Victoria and reporting the great outflow at Ripon Falls on the Lake's northern shore.
European involvement in Egypt goes back to the time of Napoleon. Laird Shipyard of Liverpool sent an iron steamer to the Nile in the 1830s. With the completion of the Suez Canal and the British takeover of Egypt in the 1870s, more British river steamers followed.
The Nile is the area's natural navigation channel, giving access to Khartoum and Sudan by steamer. The Siege of Khartoum was broken with purpose-built sternwheelers shipped from England and steamed up the river to retake the city. After this came regular steam navigation of the river. With British Forces in Egypt in the First World War and the inter-war years, river steamers provided both security and sightseeing to the Pyramids and Thebes. Steam navigation remained integral to the two countries as late as 1962. Sudan steamer traffic was a lifeline as few railways or roads were built in that country. Most paddle steamers have been retired to shorefront service, but modern diesel tourist boats remain on the river.
Modern era
The Nile has long been used to transport goods along its length. Winter winds blow south, up river, so ships could sail up river, and down river using the flow of the river. While most Egyptians still live in the Nile valley, the 1970 completion of the Aswan High Dam ended the summer floods and their renewal of the fertile soil, fundamentally changing farming practices. The Nile supports much of the population living along its banks, enabling Egyptians to live in otherwise inhospitable regions of the Sahara. The rivers's flow is disturbed at several points by the Cataracts of the Nile, which are sections of faster-flowing water with many small islands, shallow water, and rocks, which form an obstacle to navigation by boats. The Sudd wetlands in Sudan also forms a formidable navigation obstacle and impede water flow, to the extent that Sudan had once attempted to canalize (the Jonglei Canal) to bypass the swamps.[43][44]
Nile cities include Khartoum, Aswan, Luxor (Thebes), and the Giza – Cairo conurbation. The first cataract, the closest to the mouth of the river, is at Aswan, north of the Aswan Dam. This part of the river is a regular tourist route, with cruise ships and traditional wooden sailing boats known as feluccas. Many cruise ships ply the route between Luxor and Aswan, stopping at Edfu and Kom Ombo along the way. Security concerns have limited cruising on the northernmost portion for many years.
A computer simulation study to plan the economic development of the Nile was directed by H.A.W. Morrice and W.N. Allan, for the Ministry of Hydro-power of the Republic of the Sudan, during 1955–1957[45][46][47] Morrice was their Hydrological Adviser, and Allan his predecessor. M.P. Barnett directed the software development and computer operations. The calculations were enabled by accurate monthly inflow data collected for 50 years. The underlying principle was the use of over-year storage, to conserve water from rainy years for use in dry years. Irrigation, navigation and other needs were considered. Each computer run postulated a set of reservoirs and operating equations for the release of water as a function of the month and the levels upstream. The behavior that would have resulted given the inflow data was modeled. Over 600 models were run. Recommendations were made to the Sudanese authorities. The calculations were run on an IBM 650 computer. Simulation studies to design water resources are discussed further in the article on Hydrology transport models, that have been used since the 1980s to analyze water quality.
Despite the development of many reservoirs, drought during the 1980s led to widespread starvation in Ethiopia and Sudan, but Egypt was nourished by water impounded in Lake Nasser. Drought has proven to be a major cause of fatality in the Nile River basin. According to a report by the Strategic Foresight Group around 170 million people have been affected by droughts in the last century with half a million lives lost.[48] From the 70 incidents of drought which took place between 1900 and 2012, 55 incidents took place in Ethiopia, Sudan, South Sudan, Kenya and Tanzania.[48]
Water sharing dispute
The Nile's water has affected the politics of East Africa and the Horn of Africa for many decades. Countries including Uganda, Sudan, Ethiopia and Kenya have complained about Egyptian domination of its water resources. The Nile Basin Initiative promotes a peaceful cooperation among those states.[49][50]Several attempts have been made to establish agreements between the countries sharing the Nile waters. It is very difficult to have all these countries agree with each other given the self-interest of each country and their political, strategic, and social differences. On 14 May 2010 at Entebbe, Ethiopia, Rwanda, Tanzania and Uganda signed a new agreement on sharing the Nile water even though this agreement raised strong opposition from Egypt and Sudan. Ideally, such international agreements should promote equitable and efficient usage of the Nile basin's water resources. Without a better understanding about the availability of the future water resources of the Nile River, we could expect more conflicts between these countries relying on the Nile for their water supply, economic and social developments.[4]
Modern achievements and exploration
The White Nile Expedition, led by South African national Hendrik Coetzee, became the first to navigate the White Nile's entire length of approximately 3,700 kilometres (2,300 mi). The expedition began at the White Nile's source, Lake Victoria in Uganda, on January 17, 2004 and arrived safely at the Mediterranean in Rosetta, four and a half months later.[51]The Blue Nile Expedition, led by geologist Pasquale Scaturro and his partner, kayaker and documentary filmmaker Gordon Brown became the first people to descend the entire Blue Nile, from Lake Tana in Ethiopia to the beaches of Alexandria on the Mediterranean. Their approximately 5,230 kilometres (3,250 mi) journey took 114 days: from December 25, 2003 to April 28, 2004. Though their expedition included others, Brown and Scaturro were the only ones to complete the entire journey.[52] Although they descended whitewater manually the team used outboard motors for much of their journey.
On January 29, 2005 Canadian Les Jickling and New Zealander Mark Tanner completed the first human powered transit of Ethiopia's Blue Nile. Their journey of over 5,000 kilometres (3,100 mi) took five months. They recount that they paddled through two war zones, regions notorious for bandits, and were arrested at gunpoint.[53]
On April 30, 2005 a team led by South Africans Peter Meredith and Hendrik Coetzee became the first to navigate the major remote source of the White Nile, the Akagera river that starts as the Ruvyironza in Bururi Province, Burundi, and ends at Lake Victoria, Uganda.
Crossings
Crossings from Khartoum to the Mediterranean Sea
[clarification needed] The following bridges cross the Blue Nile and connect Khartoum to Khartoum North:- Mac Nimir Bridge
- Green Purple Black Red Yellow Nile Road & Railway Bridge
- Burri Bridge
- Elmansheya Bridge
- Soda bridge
- Black Nile Bridge
- Fitayhab Bridge
- Al Dabbaseen Bridge (under construction)[when?]
- Omhuraz Bridge (proposed)[citation needed]
The following bridges cross to Tuti from Khartoum states three cities
- Khartoum-tuti Bridge
- Omdurman-Tuti Suspension Bridge (proposed)[citation needed]
- Khartoum North-tuti Bridge (proposed)[citation needed]
- Shandi Bridge, Shendi
- Atbarah Bridge, Atbarah
- Merowe Dam, Merowe
- Merowe Bridge, Merowe
- Aswan Bridge, Aswan
- Luxor Bridge, Luxor
- Suhag Bridge, Suhag
- Assiut Bridge, Assiut
- Al Minya Bridge, Minya
- Al Marazeek Bridge, Helwan
- First Ring Road Bridge (Moneeb Crossing), Cairo
- Abbas Bridge, Cairo
- University Bridge, Cairo
- Qasr al-Nil Bridge, Cairo
- 6th October Bridge, Cairo
- Abu El Ela Bridge, Cairo (removed in 1998)
- New Abu El Ela Bridge, Cairo
- Imbaba Bridge, Cairo
- Rod Elfarag Bridge, Cairo
- Second Ring Road Bridge, Cairo
- Banha Bridge, Banha
- Samanoud Bridge, Samanoud
- Mansoura 2 Bridges, Mansoura
- Talkha Bridge, Talkha
- Shirbine high Bridge
- Shirbine Bridge
- Kafr Sad - Farscor Bridge
- International Coastal Road Bridge
- Damietta high Bridge, Damietta
- Damietta Bridge, Damietta
- Kafr El Zayat Bridges, Kafr El Zayat
- Zefta Bridge, Zefta
Crossings from Rwanda to Khartoum
- Nalubaale Bridge, Jinja, Uganda (Formerly Owen Falls Bridge)
- Karuma Bridge, Karuma, Uganda
- Pakwach Bridge, Uganda
Images and media of the Nile
-
Murchison Falls in Uganda, between Lake Victoria and Lake Kyoga -
Valley of the Nile near Luxor, Egypt
-
River and mountain scenery on the Nile
-
People living on the banks of the Nile