
Drive for innovation: Electric vehicles are a major target for R&D on novel battery materials. (Image courtesy: imec)
Note to Readers: This article first appeared in
the 2018 Physics World Focus on Energy Technologies Engineering a
sustainable, electrified future means developing battery materials with
properties that surpass those found in current technologies.
In recent years, Li-ion batteries have also become a staple solution in efforts to solve the interlinked conundrums of climate change and renewable energy. Increasingly, they are being used to power electric vehicles and as the principal components of home-based devices that store energy generated from renewable sources, helping to balance an increasingly diverse and smart electrical grid. The technology has improved too: over the past two and a half decades, battery experts have succeeded in making Li-ion batteries 5–10% more efficient each year, just by further optimizing the existing architecture.
Ultimately, though, getting from where we are now to a truly carbon-free economy will require better-performing batteries than today’s (or even tomorrow’s) Li-ion technology can deliver. In electric vehicles, for example, a key consideration is for batteries to be as small and lightweight as possible.
Achieving that goal calls for energy densities that are much higher than the 300 Wh/kg and 800 Wh/L which are seen as the practical limits for today’s Li-ion technology.
Another issue holding back the adoption of electric vehicles is cost, which is currently still around 300–200 $/kWh, although that is widely projected to go below 100 $/kWh by 2025 or even earlier. The time required to recharge a battery pack – still in the range of a few hours – will also have to come down, and as batteries move into economically critical applications such as grid storage and grid balancing, very long lifetimes (a decade or more) will become a key consideration too.
There is still some room left to improve existing Li-ion technology, but not enough to meet future requirements. Instead, the process of battery innovation needs a step change: materials-science breakthroughs, new electrode chemistries and architectures that have much higher energy densities, new electrolytes that can deliver the necessary high conductivity – all in a battery that remains safe and is long-lasting as well as economical and sustainable to produce.
Lithium magic
To appreciate why this is such a challenge, it helps to understand the basic architecture of existing batteries. Rechargeable Li-ion batteries are made up of one or more cells, each of which is a small chemical factory essentially consisting of two electrodes with an electrolyte in between. When the electrodes are connected (for example with a wire via a lamp), an electrochemical process begins. In the anode, electrons and lithium ions are separated, and the electrons buzz through the wire and light up the lamp. Meanwhile, the positively-charged lithium ions move through the electrolyte to the cathode. There, electrons and Li-ions combine again, but in a lower energy state than before.The beauty of rechargeable batteries is that these processes can be reversed, returning lithium ions to the anode and restoring the energy states and the original difference in electrical potential between the electrodes. Lithium ions are well suited for this task. Lithium is not only the lightest metal in the periodic table, but also the most reactive and will most easily part with its electrons. It has been chosen as the basis for rechargeable batteries precisely because it can do the most work with the least mass and the fewest chemical complications. More specifically, in batteries using lithium, it is possible to make the electric potential difference between anodes and cathodes higher than is possible with other materials.
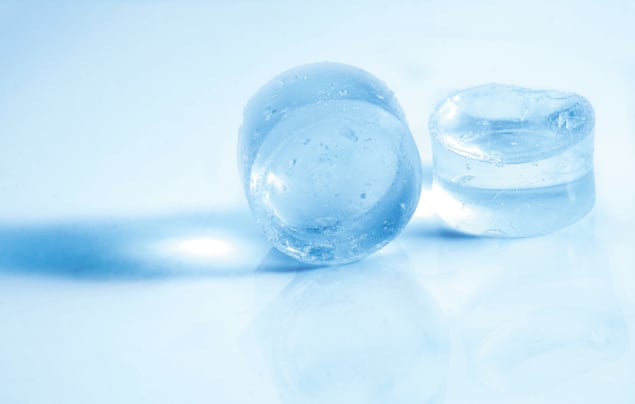
Seeking a solid electrolyte
The electrolyte in most batteries is a liquid. This allows the electrolyte not only to fill the space between the electrodes but also to soak them, completely filling all voids and spaces and providing as much contact as possible between the electrodes and the electrolyte. To complete the picture, a porous membrane is added between the electrodes. This inhibits electrical contact between the electrodes and prevents finger like outgrowths of lithium from touching and short-circuiting the battery.For all the advantages of liquid electrolytes, though, scientists have long sought to develop solid alternatives. A solid electrolyte material would eliminate several issues at the same time. Most importantly, it would replace the membrane, allowing the electrodes to be placed much closer together without touching, thereby, making the battery more compact and boosting its energy density. A solid electrolyte would also make batteries stronger, potentially meaning that the amount of protective and structural casing could be cut without compromising on safety.
Unfortunately, the solid electrolytes proposed so far have generally fallen short in one way or another. In particular, they lack the necessary conductivity (expressed in milli-Siemens per centimetre, or mS/cm). Unsurprisingly, ions tend not to move as freely through a solid as they do through a liquid. That reduces both the speed at which a battery can charge and, conversely, the quantity of power it can release in a given time.
Scientists at imec – one of Europe’s premier nanotechnology R&D centres, and a partner in the EnergyVille consortium for sustainable energy and intelligent energy systems research – recently came up with a potential solution. The new material is a nanoporous oxide mix filled with ionic compounds and other additives, with the pores giving it a surface area of about 500 m2/mL – “comparable to an Olympic swimming pool folded into a shot glass,” says Philippe Vereecken, imec’s head of battery research. Because ions move faster along the pores’ surface than in the middle of a lithium salt electrolyte, he explains, this large surface area amplifies the ionic conductivity of the nanoengineered solid. The result is a material with a conductivity of 10 mS/cm at room temperature – equivalent to today’s liquid electrolytes.
Using this new electrolyte material, imec’s engineers have built a cell prototype using standard available electrodes: LFP (LiFePO4) for the cathode and LTO (Li4Ti5O12) for the anode. While charging, the new cell reached 80% of its capacity in one hour, which is already comparable to a similar cell made with a liquid electrolyte. Vereecken adds that the team hopes for even better results with future devices. “Computations show that the new material might even be engineered to sustain conductivities of up to 100 mS/cm,” he says.
Meanwhile, back at the electrode
Electrodes are conventionally made from sintered and compressed powders. Combining these with a solid electrolyte would normally entail mixing the electrode as a powder with the electrolyte also in powder form, and then compressing the result for a maximum contact. But even then, there will always remain pores and voids that are not filled and the contact surface will be much smaller than is possible with a liquid electrolyte that fully soaks the electrode.Lithium-sulphur is a promising material that could store more energy than today’s technology allows

Imec’s new nano-composite material avoids this problem because it is actually applied as a liquid, via wet chemical coating, and only afterwards converted into a solid. That way it can impregnate dense powder electrodes, filling all cavities and making maximum contact just as a liquid electrolyte would. Another benefit is that even as a solid, the material remains somewhat elastic, which is essential as some electrodes expand and contract during battery charging and discharging. A final advantage is that because the solid material can be applied via a wet precursor, it is compatible with current Li-ion battery fabrication processes – something that Vereecken says is “quite important for the battery manufacturers” because otherwise more “disruptive” fabrication processes would have to be put in place.
To arrive at the energy densities required to give electric vehicles a long driving range, though, still more changes are needed. One possibility is to make the particles in the electrode powders smaller, so that they can be packed more densely. This would produce a larger contact surface with the electrolyte per volume, improving the energy density and charging rate of the cell. There is a catch, though: while a larger contact surface results in more ions being created and changing sides within the battery, it also gives more way for unwanted reactions that will degrade the battery’s materials and shorten its lifetime. “To improve the stability,” says Vereecken, “imec’s experts work on a solution where they coat all particles with an ultrathin buffer layer.” The challenge, he says, is to make these layers both chemically inert and highly conductive.
Introducing new materials
By combining solid electrolytes with thicker electrodes made from smaller particles, it may be possible to produce batteries with energy densities that exceed the current maximum of around 800 Wh/L. These batteries could also charge in 30 minutes or less. But to extend the energy density even further, to 1000 Wh/L and beyond, a worldwide effort is on to look for new and better electrode materials. Anodes, for example, are currently made from carbon in the form of graphite. That carbon could be replaced by silicon, which can hold up to ten times as many lithium ions per gram of electrode. The drawback is that when the battery is charged, a silicon anode will expand to more than three times its normal size as it fills with lithium ions. This may break up the electrode, and possibly even the battery casing.A better alternative may be to replace carbon with pure lithium metal. A lithium anode will also store up to ten times as much lithium ions per gram of electrode as graphite, but without the swelling seen in silicon anodes. Lithium anodes were, in fact, used in the early days of Li-ion batteries, but as the metal is very reactive, especially in combination with liquid electrolytes, the idea was dropped in favour of more stable alternatives. Vereecken, however, believes that progress in solid electrolytes means it is “high time to revisit lithium metal as a material for the anode”, especially since it is possible to add protective functional coatings to nanoparticles.
Disruptive innovations are on the horizon for cathodes as well. Lithium-sulphur, for example, is a promising material that could store more energy than today’s technology allows. Indeed, the “ideal” lithium battery might well feature a lithium-air (lithium peroxide) cathode in combination with a pure lithium anode. But whereas the material composition of these batteries sounds simple, the path to realizing them will not be so easy, and there is still some way to go before any of these developments will be integrated into commercial batteries. Once that happens, though, huge payoffs are possible. The most obvious would be electrical cars that drive farther and charge faster, but better lithium batteries could also be the breakthrough needed to make renewable power ubiquitous – and thus finally let us off the fossil-fuel hook.