Nonmetals in their periodic table context |
![]() |
|
usually/always counted as a nonmetal |
sometimes counted as a nonmetal |
At astatine's status is not clear. While usually counted as nonmetallic, relativistic effects suggest it may be a metal. |
Cn Fl Og copernicium, flerovium, and/or oganesson may turn out to be nonmetallic however their status has not been confirmed. |
A nonmetal is a chemical element that mostly lacks metallic properties. Seventeen elements are generally considered nonmetals, though some authors recognize more or fewer depending on the properties considered most representative of metallic or nonmetallic character. Some borderline elements further complicate the situation.
Nonmetals tend to have low density and high electronegativity (the ability of an atom in a molecule to attract electrons to itself). They range from colorless gases like hydrogen to shiny solids like the graphite form of carbon. Nonmetals are often poor conductors of heat and electricity, and when solid tend to be brittle or crumbly. In contrast, metals are good conductors and most are pliable. While compounds of metals tend to be basic, those of nonmetals tend to be acidic.
The two lightest nonmetals, hydrogen and helium, together make up about 98% of the observable ordinary matter in the universe by mass. Five nonmetallic elements—hydrogen, carbon, nitrogen, oxygen, and silicon—make up the overwhelming majority of the Earth's crust, atmosphere, oceans and biosphere.
The distinct properties of nonmetallic elements allow for specific uses that metals often cannot achieve. Elements like hydrogen, oxygen, carbon, and nitrogen are essential building blocks for life itself. Moreover, nonmetallic elements are integral to industries such as electronics, energy storage, agriculture, and chemical production.
Most nonmetallic elements were not identified until the 18th and 19th centuries. While a distinction between metals and other minerals had existed since antiquity, a basic classification of chemical elements as metallic or nonmetallic emerged only in the late 18th century. Since then nigh on two dozen properties have been suggested as single criteria for distinguishing nonmetals from metals.
Definition and applicable elements
- Properties mentioned hereafter refer to the elements in their most stable forms in ambient conditions unless otherwise mentioned

Nonmetal chemical element generally have low density and high electronegativity. They lack most or all of the properties commonly associated with metals: shininess; malleability and ductility; good thermal and electrical conductivity; and a capacity to (mostly) produce basic oxides when combined with oxygen.
There is no precise definition of a nonmetal; any list of such is open to debate and revision. Which elements are included depends on the properties regarded as most representative of nonmetallic or metallic character.
These fourteen elements are effectively always recognized as nonmetals:
Hydrogen, Nitrogen, Oxygen, Sulfur Fluorine, Chlorine, Bromine, Iodine Helium, Neon, Argon, Krypton, Xenon, Radon
Three more are commonly called nonmetals, but some sources list them as metalloids:
The six elements most commonly recognized as metalloids, which are typically seen as intermediates between metals and nonmetals, are here counted as a type of nonmetal due to their relatively low densities and predominantly nonmetallic chemistry, and for comparative purposes:
Of the 118 known elements, roughly 20% are classified as nonmetals. Opinions differ as to the status of astatine. Its rarity and extreme radioactivity has resulted in it being frequently ignored in the literature. A comprehensive understanding of its properties is lacking and its classification remains uncertain. As a halogen it has usually been presumed to be a nonmetal. Chemically, studies on trace quantities of astatine, which are not necessarily reliable, have demonstrated characteristics of both metals and nonmetals. Alternatively, given the near-metallic character of its lighter congener iodine, a succession of authors have speculated or presumed astatine would be a metal. A 2013 study based on relativistic chemistry concluded that it would be a monatomic metal with a close-packed crystalline structure, although this has not been experimentally verified. Astatine is precluded from further consideration in this article due to uncertainty as to its behavior and status. The superheavy elements copernicium (element 112), flerovium (114), and oganesson (118) may or may not turn out to be nonmetals; their status has not been confirmed.
General properties
Physical
About half of nonmetallic elements are gases; most of the rest are shiny solids. Bromine, the only liquid, is so volatile that it is usually topped by a layer of its fumes; sulfur is the only colored solid nonmetal. The gaseous and liquid nonmetals have very low densities, melting and boiling points, and are poor conductors of heat and electricity. The solid elements have low densities and low mechanical and structural strength (being brittle or crumbly), but a wide range of electrical conductivity.
These diverse forms are caused by varied internal structures and bonding arrangements. Nonmetals existing as discrete atoms like xenon, or as small molecules, such as oxygen, sulfur, and bromine, have low melting and boiling points; many are gases at room temperature, as they are held together by weak London dispersion forces acting between their atoms or molecules. In contrast, nonmetals that form giant structures, such as chains of up to 1,000 atoms (e.g., selenium), sheets (e.g., carbon as graphite), or three-dimensional lattices (e.g., silicon) have higher melting and boiling points, and are all solids, as it takes more energy to overcome their stronger covalent bonds. Nonmetals closer to the left or bottom of the periodic table, often have some weak metallic interactions between their molecules, chains, or layers, consistent with their proximity to the metals; this occurs in boron, carbon, phosphorus, arsenic, selenium, antimony, tellurium and iodine.
The structures of nonmetallic elements differ from those of metals primarily due to variations in valence electrons and atomic size. Metals typically have fewer valence electrons than available orbitals, leading them to share electrons with many nearby atoms, resulting in centrosymmetrical crystalline structures. In contrast, nonmetals share only the electrons required to achieve a noble gas electron configuration. For example, nitrogen forms diatomic molecules featuring a triple bonds between each atom, both of which thereby attain the configuration of the noble gas neon; while antimony's larger atomic size prevents triple bonding, resulting in buckled layers in which each antimony atom is singly bonded with three other nearby atoms.
Nonmetals vary greatly in appearance. The shininess of boron, graphitic carbon, silicon, black phosphorus, germanium, arsenic, selenium, antimony, tellurium, and iodine is a result of their structures featuring varying degrees of delocalized (free-moving) electrons that scatter incoming visible light. The colored nonmetals (sulfur, fluorine, chlorine, bromine) absorb some colors (wavelengths) and transmit the complementary or opposite colors. For example, chlorine’s "familiar yellow-green colour ... is due to a broad region of absorption in the violet and blue regions of the spectrum". For the colorless nonmetals (hydrogen, nitrogen, oxygen, and the noble gases), their electrons are held sufficiently strongly so that no absorption happens in the visible part of the spectrum, and all visible light is transmitted.
The electrical and thermal conductivities of nonmetals, along with the brittle nature of solid nonmetals are likewise related to their internal arrangements. Whereas good conductivity and plasticity (malleability, ductility) are ordinarily associated with the presence of free-moving and evenly distributed electrons in metals, the electrons in nonmetals typically lack such mobility. Among nonmetallic elements, good electrical and thermal conductivity is seen only in carbon (as graphite, along its planes), arsenic, and antimony. Good thermal conductivity otherwise occurs only in boron, silicon, phosphorus, and germanium; such conductivity is transmitted though vibrations of the crystalline lattices of these elements. Moderate electrical conductivity is observed in boron, silicon, phosphorus, germanium, selenium, tellurium, and iodine. Plasticity occurs under limited circumstances in carbon, as seen in exfoliated (expanded) graphite and carbon nanotube wire, in white phosphorus (soft as wax, pliable and can be cut with a knife, at room temperature), in plastic sulfur, and in selenium which can be drawn into wires from its molten state.
The physical differences between metals and nonmetals arise from internal and external atomic forces. Internally, the positive charge stemming from the protons in an atom's nucleus acts to hold the atom's outer electrons in place. Externally, the same electrons are subject to attractive forces from protons in neighboring atoms. When the external forces are greater than, or equal to, the internal force, the outer electrons are expected to become relatively free to move between atoms, and metallic properties are predicted. Otherwise nonmetallic properties are expected.
Allotropes
Aspect | Metals | Nonmetals |
---|---|---|
Electronegativity | Lower than nonmetals, with some exceptions |
Relatively high |
Chemical bonding | ||
Seldom form covalent bonds |
Frequently form covalent bonds | |
Metallic bonds (alloys) between metals |
Covalent bonds between nonmetals | |
Ionic bonds between nonmetals and metals | ||
Oxidation states |
Positive | Negative or positive |
Oxides | Basic in lower oxides; increasingly acidic in higher oxides |
Acidic; never basic |
In aqueous solution[63] |
Exist as cations | Exist as anions or oxyanions |
Many nonmetallic elements exhibit a range of allotropic forms, each with distinct physical properties that may vary between metallic and nonmetallic. For example, carbon, a versatile nonmetal, can manifest as graphite, diamond, and other forms, with graphite displaying relatively good electrical conductivity, while diamond is transparent and an extremely poor conductor of electricity. Carbon further exists in several allotropic structures, including buckminsterfullerene, and amorphous and paracrystalline (mixed amorphous and crystalline) variations. Allotropes also occur for the other unclassified nonmetals, the metalloids, and iodine among the halogen nonmetals.
Chemical
Nonmetals possess relatively high values of electronegativity and tend to form acidic compounds. For example, solid nonmetals (including metalloids) react with nitric acid to produce either an acid, or an oxide with predominately acidic properties.
They tend to gain or share electrons during chemical reactions, in contrast to metals which tend to donate electrons. This behavior is closely related to the stability of electron configurations in the noble gases, which have complete outer shells. Nonmetals generally gain enough electrons to attain the electron configuration of the following noble gas, while metals tend to lose electrons, achieving the electron configuration of the preceding noble gas. These tendencies in nonmetallic elements are succinctly summarized by the duet and octet rules of thumb. Metals, on the other hand, follow a less rigorously predictive 18-electron rule.
Furthermore, nonmetals typically exhibit higher ionization energies, electron affinities, and standard reduction potentials than metals. General, the higher these values are (including electronegativity) the more nonmetallic the element tends to be.
The chemical distinctions between metals and nonmetals primarily stem from the attractive force between the positive nuclear charge of an individual atom and its negatively charged outer electrons. From left to right across each period of the periodic table, the nuclear charge increases in tandem with the number of protons in the atomic nucleus. Consequently, there is a corresponding reduction in atomic radius as the heightened nuclear charge draws the outer electrons closer to the nucleus core. In metals, the impact of the nuclear charge is generally weaker compared to nonmetallic elements. As a result, in chemical bonding, metals tend to lose electrons, leading to the formation of positively charged or polarized atoms or ions, while nonmetals tend to gain these electrons due to their stronger nuclear charge, resulting in negatively charged ions or polarized atoms.
The number of compounds formed by nonmetals is vast. The first 10 places in a "top 20" table of elements most frequently encountered in 895,501,834 compounds, as listed in the Chemical Abstracts Service
register for November 2, 2021, were occupied by nonmetals. Hydrogen,
carbon, oxygen, and nitrogen collectively appeared in most (80%) of
compounds. Silicon, a metalloid, ranked 11th. The highest-rated metal,
with an occurrence frequency of 0.14%, was iron, in 12th place. A few examples of nonmetal compounds are: boric acid (H
3BO
3), used in ceramic glazes; selenocysteine (C
3H
7NO
2Se), the 21st amino acid of life; phosphorus sesquisulfide (P4S3), found in strike anywhere matches; and teflon ((C
2F
4)n), used to create non-stick coatings for pans and other cookware.
Complications


Adding complexity to the chemistry of the nonmetals are the anomalies observed in the first row of each periodic table block. These anomalies are especially prominent in hydrogen, boron (whether as a nonmetal or metalloid), carbon, nitrogen, oxygen, and fluorine. In later rows these anomalies manifest as secondary periodicity or non-uniform periodic trends within most of the p-block groups, and unusual oxidation states in the heavier nonmetals.
First row anomaly
Starting with hydrogen, the first row anomaly primarily arises from the electron configurations of the elements concerned. Hydrogen is particularly notable for its diverse bonding behaviors. It most commonly forms covalent bonds, but it can also lose its single electron in an aqueous solution, leaving behind a bare proton with tremendous polarizing power. Consequently, this proton can attach itself to the lone electron pair of an oxygen atom in a water molecule, laying the foundation for acid-base chemistry. Moreover, a hydrogen atom in a molecule can form a second, albeit weaker, bond with an atom or group of atoms in another molecule. As Cressey explains, such bonding, "helps give snowflakes their hexagonal symmetry, binds DNA into a double helix; shapes the three-dimensional forms of proteins; and even raises water's boiling point high enough to make a decent cup of tea."
Hydrogen and helium, as well as boron through neon, have unusually small atomic radii. This phenomenon arises because the 1s and 2p subshells lack inner analogues (meaning there is no zero shell and no 1p subshell), and they therefore experience no electron repulsion effects, unlike the 3p, 4p, and 5p subshells of heavier elements. A a result, ionization energies and electronegativities among these elements are higher than what periodic trends would otherwise suggest. The compact atomic radii of carbon, nitrogen, and oxygen facilitate the formation of double or triple bonds.
While it would normally be expected, on electron configuration consistency grounds, that hydrogen and helium would be placed atop the s-block elements, the significant first row anomaly shown by these two elements justifies alternative placements. Hydrogen is occasionally positioned above fluorine, in group 17, rather than above lithium in group 1. Helium is commonly placed above neon, in group 18, rather than above beryllium in group 2.
Secondary periodicity
An alternation in certain periodic trends becomes evident when descending groups 13 to 15, and to a lesser extent, groups 16 and 17. Immediately after the first row of d-block metals, from scandium to zinc, the 3d electrons in the p-block elements—specifically, gallium (a metal), germanium, arsenic, selenium, and bromine—prove less effective at shielding the increasing positive nuclear charge. This same effect is observed with the emergence of fourteen f-block metals located between barium and lutetium, ultimately leading to atomic radii that are smaller than expected for elements from hafnium (Hf) onward.
Unusual oxidation states
The larger atomic radii of the heavier group 15–18 nonmetals enable higher bulk coordination numbers, and result in lower electronegativity values that better tolerate higher positive charges. The elements involved are thereby able to exhibit oxidation states other than the lowest for their group (that is, 3, 2, 1, or 0), for example in phosphorus pentachloride (PCl5), sulfur hexafluoride (SF6), iodine heptafluoride (IF7), and xenon difluoride (XeF2).
Types
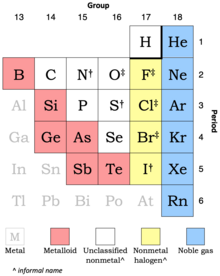
† moderately strong oxidizing agents
‡ strong oxidizing agents
The classification of nonmetals can vary, with approaches ranging from as few as two types to as many as six or seven. For instance, the periodic table in the Encyclopaedia Britannica recognizes noble gases, halogens, and other nonmetals, and splits the elements commonly recognized as metalloids between "other metals" and "other nonmetals". On the other hand, the Royal Society of Chemistry periodic table uses different colors for each of its eight main groups, with nonmetals represented in seven of these.
When traversing the periodic table from right to left, three or four types of nonmetals are more or less commonly discerned:
- the relatively inert noble gases;
- a set of chemically strong halogen elements—fluorine, chlorine, bromine and iodine—sometimes referred to as nonmetal halogens or halogen nonmetals (as used here) or stable halogens;
- a set of unclassified nonmetals, encompassing elements like hydrogen, carbon, nitrogen, and oxygen, for which there is no widely recognized collective name; and
- the chemically weak nonmetallic metalloids which are sometimes considered nonmetals and sometimes considered a third category distinct from metals and nonmetals.
Since metalloids occupy a transition region or "frontier territory", where metals meet nonmetals, their classification varies among authors. Some consider them distinct from both metals and nonmetals, while others classify them as nonmetals. There are also authors who categorize certain metalloids as metals, such as arsenic and antimony, due to their similarities to heavy metals. In this context metalloids are here treated as nonmetals, based on their relatively low densities, high electronegativity, and chemical behavior, and for comparative purposes.
Aside from the metalloids, some boundary fuzziness and overlapping (as occurs with classification schemes generally), can be discerned among the other types of nonmetals. Carbon, phosphorus, selenium, and iodine border the metalloids and show some metallic character, as does hydrogen. Among the noble gases, radon is the most metallic and begins to show some cationic behavior, which is unusual for a nonmetal.
Noble gases

Six nonmetals are classified as noble gases: helium, neon, argon, krypton, xenon, and the radioactive radon. In conventional periodic tables they occupy the rightmost column. They are called noble gases due to their exceptionally low chemical reactivity.
These elements exhibit remarkably similar properties, characterized by their colorlessness, odorlessness, and nonflammability. Due to their closed outer electron shells, noble gases possess feeble interatomic forces of attraction, leading to exceptionally low melting and boiling points. As a consequence, they all exist as gases under standard conditions, even those with atomic masses surpassing many typically solid elements.
Chemically, the noble gases exhibit relatively high ionization energies, negligible or negative electron affinities, and high to very high electronegativities. The number of compounds formed by noble gases is in the hundreds and continues to expand, with most of these compounds involving the combination of oxygen or fluorine with either krypton, xenon, or radon.
An analogy between the noble gases and noble metals (such as platinum and gold) is commonly drawn, as they share a similar reluctance to combine with other elements. As a further analogy, xenon, in the +8 oxidation state, forms a pale yellow explosive oxide, XeO4, while osmium, another noble metal, forms a yellow, strongly oxidizing oxide, OsO4. Additionally, there are parallels in the formulas of the oxyfluorides: XeO2F4 and OsO2F4, and XeO3F2 and OsO3F2.
About 1015 tonnes of noble gases are present in the Earth's atmosphere. Additionally, natural gas can contain as much as 7% helium. Radon diffuses out of rocks, where it forms during the natural decay sequence of uranium and thorium. In the Earth's core there may be around 1013 tons of xenon, in the form of stable XeFe3 and XeNi3 intermetallic compounds. This could explain why "studies of the Earth's atmosphere have shown that more than 90% of the expected amount of Xe is depleted."
Halogen nonmetals
Corrosive chlorine, a halogen nonmetal, combines with highly reactive sodium to form stable, unreactive table salt.
Although the halogen nonmetals are notably reactive and corrosive elements, when combined with the equally reactive alkali metals, they form relatively stable and unreactive chemical salts found in everyday toothpaste (NaF); table salt (NaCl); swimming pool disinfectant (NaBr); or the food supplement (KI). The term "halogen" itself means "salt former".
Physically, fluorine and chlorine exist as pale yellow and yellowish-green gases, respectively, while bromine is a reddish-brown liquid, typically covered by a layer of its fumes; iodine, when observed under white light, appears as a metallic-looking solid. Electrically, the first three elements function as insulators while iodine behaves as a semiconductor (along its planes).
Chemically, the halogen nonmetals exhibit high ionization energies, electron affinities, and electronegativity values, and are mostly relatively strong oxidizing agents. These characteristics contribute to their corrosive nature. All four elements tend to form primarily ionic compounds with metals, in contrast to the remaining nonmetals (except for oxygen) which tend to form primarily covalent compounds with metals. The highly reactive and strongly electronegative nature of the halogen nonmetals epitomizes nonmetallic character.
The highly nonmetallic halogens in group 17 find their counterparts in the highly reactive alkali metals, such as sodium and potassium, in group 1. Further, and like the halogen nonmetals, most of the alkali metals are known to form −1 anions, a characteristic seldom observed among metals.
The halogen nonmetals are commonly found in salt-related minerals. Fluorine, for instance, is present in fluorite (CaF2), a mineral found widely. Chlorine, bromine, and iodine are typically found in brines. Exceptionally, a study reported in 2012 noted the presence of 0.04% native fluorine (F
2) by weight in antozonite, attributing these inclusions to radiation from tiny amounts of uranium.
Metalloids
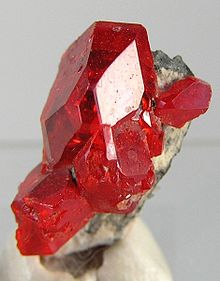
The six elements more commonly recognized as metalloids are boron, silicon, germanium, arsenic, antimony, and tellurium, all of which have a metallic appearance. (There are other elements less commonly recongised as metalloids, including carbon, aluminium, selenium and polonium. They have metallic and nonmetallic properties, but one or the other kind predominates.) On a standard periodic table, they occupy a diagonal region within the p-block extending from boron at the upper left to tellurium at the lower right, along the dividing line between metals and nonmetals shown on some tables.
They are brittle and poor-to-good conductors of heat and electricity. Specifically, boron, silicon, germanium, and tellurium are semiconductors. Arsenic and antimony have the electronic structures of semimetals, although both have less stable semiconducting forms.
Chemically, metalloids generally behave like (weak) nonmetals. Among the nonmetallic elements they tend to have the lowest ionization energies, electron affinities, and electronegativity values, and are relatively weak oxidizing agents. Additionally, they tend to form alloys when combined with metals.
In periodic table geography terms, while metalloids are usually regarded as elements located between metals and nonmetals, they are occasionally compared to the post-transition metals, immediately to their left. The latter are an indeterminate set of weakly metallic elements including tin, lead and bismuth. Dingle explains the situation this way:
... with "no-doubt" metals on the far left of the table, and no-doubt non-metals on the far right ... the gap between the two extremes is bridged first by the poor (post-transition) metals, and then by the metalloids—which, perhaps by the same token, might collectively be renamed the "poor non-metals".
The metalloids are commonly found combined with oxygen, sulfur, or, in the case of tellurium, gold or silver. Boron is typically found in boron-oxygen borate minerals, including in volcanic spring waters. Silicon is present in the silicon-oxygen mineral silica (sand). Germanium, arsenic, and antimony are mainly found as components of sulfide ores. Tellurium is often found in telluride minerals of gold or silver. In some instances, native forms of arsenic, antimony, and tellurium have been reported.
Unclassified nonmetals

After classifying the nonmetallic elements into noble gases, halogens, and metalloids, the remaining seven nonmetals are hydrogen, carbon, nitrogen, oxygen, phosphorus, sulfur, and selenium.
In their most stable forms, three of these are colorless gases (H, N, O); three have a metal-like appearance (C, P, Se); and one appears yellow (S). Electrically, graphitic carbon behaves as a semimetal along its planes and a semiconductor perpendicular to its planes; phosphorus and selenium are semiconductors; while hydrogen, nitrogen, oxygen, and sulfur are insulators.
These elements are often considered too diverse to merit a collective classification, and have been referred to as other nonmetals, or simply as nonmetals, located between the metalloids and the halogens. As a result, their chemistry is typically taught disparately, according to their respective periodic table groups: hydrogen in group 1; the group 14 nonmetals (including carbon, and possibly silicon and germanium); the group 15 nonmetals (including nitrogen, phosphorus, and possibly arsenic and antimony); and the group 16 nonmetals (including oxygen, sulfur, selenium, and possibly tellurium). Authors may choose other subdivisions based on their preferences.
Hydrogen, in particular, behaves in some respects like a metal and in others like a nonmetal. Like a metal it can, for example, form a solvated cation in aqueous solution; it can substitute for alkali metals in compounds such as the chlorides (NaCl cf. HCl) and nitrates (KNO3 cf. HNO3), and in certain alkali metal organometallic structures; and it can form alloy-like hydrides with some transition metals. Conversely, it is an insulating diatomic gas, akin to the nonmetals nitrogen, oxygen, fluorine and chlorine. In chemical reactions, it tends to ultimately attain the electron configuration of helium (the following noble gas) behaving in this way as a nonmetal. It attains this configuration by forming a covalent or ionic bond or, if it has initially given up its electron, by attaching itself to a lone pair of electrons.
Some or all of these nonmetals share several properties. Being less reactive than the halogens, they can occur naturally in the environment. They have significant roles in biology and geochemistry. Collectively, their physical and chemical characteristics can be described as "moderately non-metallic". However, they all have corrosive aspects. Hydrogen can corrode metals. Carbon corrosion can occur in fuel cells. Acid rain is caused by dissolved nitrogen or sulfur. Oxygen causes iron to corrode via rust. White phosphorus, the most unstable form, ignites in air and leaves behind phosphoric acid residue. Untreated selenium in soils can lead to the formation of corrosive hydrogen selenide gas. When combined with metals, the unclassified nonmetals can form high-hardness (interstitial or refractory) compounds due to their relatively small atomic radii and sufficiently low ionization energies. They also exhibit a tendency to bond to themselves, particularly in solid compounds. Additionally, diagonal periodic table relationships among these nonmetals mirror similar relationships among the metalloids.
In terms of the geography of the periodic table, the location of the bulk of the unclassified nonmetals can be compared to that of the transition metals. The unclassified nonmetals are positioned between the highly reactive halogens on the right and the weakly nonmetallic metalloids on the left. The transition metals occupy a region between the reactive alkali and alkaline earth metals on the left side of the periodic table, and the "poor" or post-transition metals on the right.
Unclassified nonmetals are typically found in elemental forms or in association with other elements:
- Hydrogen is present in the world's oceans as a component of water and occurs in natural gas as a component of methane and hydrogen sulfide.
- Carbon can be found in limestone, dolomite, and marble, as carbonates. Additionally, carbon exists as graphite, primarily occurring in metamorphic silicate rocks, resulting from the compression and heating of sedimentary carbon compounds.
- Oxygen is found in the atmosphere; in the oceans as a component of water; and in the Earth's crust as oxide minerals.
- Phosphorus minerals are widespread, typically appearing as phosphorus-oxygen phosphates.
- Elemental sulfur can be found in or near hot springs and volcanic regions around the world, while sulfur minerals are common and are often found as sulfides or oxygen-sulfur sulfates.
- Selenium is found in metal sulfide ores, where it may partially replace sulfur. In rare instances, elemental selenium can also be found.
Abundance, extraction, and uses
Domain | Main components | Next most abundant |
---|---|---|
Universe | H 70.5%, He 27.5% | O 1% |
Atmosphere | N 78%, O 21% | Ar 0.5% |
Hydrosphere | O 66.2%, H 33.2% | Cl 0.3% |
Biomass | O 63%, C 20%, H 10% | N 3.0% |
Crust | O 61%, Si 20% | H 2.9% |
![]() |
Nearly all nonmetals have uses in: Household goods, lighting and lasers, and medicine and pharmaceuticals |
Most nonmetals have uses in: Agrochemicals and dyestuffs |
Some nonmetals have uses in or as: Alloys, cryogenics and refrigerants, explosives, fire retardants, fuel cells, inert air replacements, insulation, mineral acids, nuclear control rods, photography, plastics, plug-in hybrid vehicles, smart phones, solar cells, water treatment, welding gases, and vulcanization |
Metalloids have uses in: Alloys, ceramics, oxide glasses, solar cells, and semiconductors |
Abundance
Hydrogen and helium dominate the universe, making up an estimated 98% of all ordinary matter by mass. Oxygen, the next most abundant element, constitutes around 1% of the universe's composition.
Five nonmetals—hydrogen, carbon, nitrogen, oxygen, and silicon—dominate the accessible structure of the earth, forming the vast majority of the Earth's crust, atmosphere, hydrosphere, and biomass as shown in the accompanying table.
Extraction
Nonmetals and metalloids are extracted in their raw forms from:
- seawater brine—chlorine, bromine, iodine;
- liquid air—nitrogen, oxygen, neon, argon, krypton, xenon;
- mineral ores—boron (borate minerals); carbon (coal; diamond; graphite); fluorine (fluorite); silicon (silica); phosphorus (phosphates); antimony (stibnite, tetrahedrite); iodine (in sodium iodate and sodium iodide);
- natural gas—hydrogen (methane), helium, sulfur (hydrogen sulfide); and
- mining byproducts—germanium (zinc ores); arsenic (copper and lead ores); selenium and tellurium (copper ores); and radon (uranium-bearing ores).
Uses
Nonmetallic elements have distinct properties that enable a wide range of natural and technological uses. In living organisms, hydrogen, oxygen, carbon, and nitrogen serve as the foundational building blocks of life. Some key technological uses of nonmetallic elements are in lighting and lasers, medicine and pharmaceuticals, and ceramics and plastics. The accompanying table groups nonmetallic elements according to their uses.
Some specific uses of later-discovered or rarer nonmetallic elements include:
- Boron, first produced in a pure form in 1909, is used in the form of high-strength fibers for aerospace components and certain sporting goods. It is also added to steel alloys to improve hardenability.
- Black phosphorus, first reported in 1916, is employed mainly in high-performance electronic devices, including field-effect transistors (FETs), owing to its exceptional charge carrier mobility. It also has potenital applications in photodetectors, optoelectronic devices, advanced solar cells and thermoelectric materials.
- Germanium, thought to be a metal up until the 1930s, was historically used in electronics, particularly early transistors and diodes, and still has roles in specialized high-frequency electronics. It is also used in the production of infrared optical components for thermal imaging and spectroscopy.
- Xenon, one of the rarest elements on Earth, finds use in high-intensity discharge lamps for bright white light in automotive headlights and marine lighting. Additionally, it serves as a contrast agent in medical imaging techniques like xenon computed tomography and xenon-enhanced magnetic resonance imaging. In space exploration, xenon is a propellant for ion thrusters, known for their efficiency.
- Radon, rarest of the noble gases, was formerly used in radiography and radiation therapy. Usually, radium in either an aqueous solution or as a porous solid was stored in a glass vessel. The radium decayed to produce radon, which was pumped off, filtered, and compressed into a small tube every few days. The tube was then sealed and removed. It was a source of gamma rays which came from bismuth-214, one of radon’s decay products. Radon has now been replaced by sources of 137Cs, 192Ir, and 103Pd.
History, background, and taxonomy
Discovery

Most nonmetallic elements were identified during the 18th and 19th centuries. However, a few nonmetals were recognized in ancient times and later historical periods. Carbon, sulfur, and antimony were among the early nonmetals known to humanity. The discovery of arsenic can be traced back to the Middle Ages, credited to the work of Albertus Magnus. A significant moment in the history of nonmetal discovery occurred in 1669 when Hennig Brand successfully isolated phosphorus from urine. Helium, identified in 1868, holds a unique distinction as the only element not initially discovered on Earth itself. Radon is the most recently identified nonmetal, with its detection occurring at the end of the 19th century.
The isolation of nonmetallic elements depended on a range of chemical and physical techniques. These methods encompassed spectroscopy, fractional distillation, radiation detection, electrolysis, ore acidification, displacement reactions, combustion, and controlled heating processes. While some nonmetals were naturally occurring as free elements, others required intricate extraction procedures:
- The noble gases, renowned for their low reactivity, were first identified via spectroscopy, air fractionation, and radioactive decay studies. Helium was initially detected by its distinctive yellow line in the solar corona spectrum. Subsequently, it was observed escaping as bubbles when uranite UO2 was dissolved in acid. Neon, argon, krypton, and xenon were obtained through the fractional distillation of air. The discovery of radon occurred three years after Henri Becquerel's pioneering research on radiation in 1896.
- The isolation of halogen nonmetals from their halides involved techniques including electrolysis, acid addition, or displacement. These efforts were not without peril, as some chemists tragically lost their lives in their pursuit of isolating fluorine.
- The unclassified nonmetals have a diverse history. Hydrogen was discovered and first described in 1671 as the product of the reaction between iron filings and dilute acids. Carbon was found naturally in forms like charcoal, soot, graphite, and diamond. Nitrogen was discovered by examining air after carefully removing oxygen. Oxygen itself was obtained by heating mercurous oxide. Phosphorus was derived from the heating of ammonium sodium hydrogen phosphate (Na(NH4)HPO4), a compound found in urine. Sulfur occurred naturally as a free element, simplifying its isolation. Selenium, was first identified as a residue in sulfuric acid.
- Metalloids were commonly isolated by heating of their oxides (boron, silicon, arsenic, tellurium) or a sulfide (germanium). Antimony was obtained primarily through the heating of its sulfide, stibnite; it was later discovered in native form.
Origin and use of the term

Although a distinction had existed between metals and other mineral substances since ancient times, it was only towards the end of the 18th century that a basic classification of chemical elements as either metallic or nonmetallic substances began to emerge. It would take another nine decades before the term "nonmetal" was widely adopted.
Around the year 340 BCE, in Book III of his treatise Meteorology, the ancient Greek philosopher Aristotle categorized substances found within the Earth into two distinct groups: metals and "fossiles". The latter category included various minerals such as realgar, ochre, ruddle, sulfur, cinnabar, and other substances that he referred to as "stones which cannot be melted".
Up until the Middle Ages the classification of minerals remained largely unchanged, albeit with varying terminology. In the fourteenth century, an English alchemist named Richardus Anglicus expanded upon the classification of minerals in his work Correctorium Alchemiae. In this text, he proposed the existence of two primary types of minerals. The first category, which he referred to as "major minerals", included well-known metals such as gold, silver, copper, tin, lead, and iron. On the other hand, the second category, labeled as "minor minerals", encompassed substances like salts, atramenta (iron sulfate), alums, vitriol, arsenic, orpiment, sulfur, and similar substances that were not metallic bodies.
The term "nonmetallic" has historical origins dating back to at least the 16th century. In a 1566 medical treatise, the French physician Loys de L'Aunay discussed the distinct properties exhibited by substances derived from plant sources. In his writings, he made a significant comparison between the characteristics of materials originating from what he referred to as metallic soils and non-metallic soils.
Later, the French chemist Nicolas Lémery discussed metallic minerals and nonmetallic minerals in his work Universal Treatise on Simple Drugs, Arranged Alphabetically published in 1699. In his writings, he contemplated whether the substance "cadmia" belonged to either the first category, akin to cobaltum (cobaltite), or the second category, exemplified by what was then known as calamine—a mixed ore containing zinc carbonate and silicate.
The pivotal moment in the systematic classification of chemical elements, distinguishing between metallic and nonmetallic substances, came in 1789 with the groundbreaking work of Antoine Lavoisier. Lavoisier, a French chemist, published the first modern list of chemical elements in his revolutionary work Traité élémentaire de chimie. In this work he categorized elements into distinct groups, including gases, metallic substances, nonmetallic substances, and earths (heat-resistant oxides). Lavoisier's work gained widespread recognition and was republished in twenty-three editions across six languages within its first seventeen years, significantly advancing the understanding of chemistry in Europe and America.
The eventual and widespread adoption of the term "nonmetal" followed a complex and lengthy developmental process that spanned nearly nine decades. In 1811, the Swedish chemist Berzelius introduced the term "metalloids" to describe nonmetallic elements, noting their ability to form negatively charged ions with oxygen in aqueous solutions. While Berzelius' terminology gained significant acceptance, it later faced criticism from some who found it counterintuitive, misapplied, or even invalid. In 1864, reports indicated that the term "metalloids" was still endorsed by leading authorities, but there were reservations about its appropriateness. The idea of designating elements like arsenic as metalloids had been considered. By as early as 1866, some authors began preferring the term "nonmetal" over "metalloid" to describe nonmetallic elements. In 1875, Kemshead observed that elements were categorized into two groups: non-metals (or metalloids) and metals. He noted that the term "non-metal", despite its compound nature, was more precise and had become universally accepted as the nomenclature of choice.
Suggested distinguishing criteria
Physical |
|
Chemical | Electron-related |
In 1809, the British chemist and inventor Humphry Davy made a groundbreaking discovery that reshaped the understanding of metals and nonmetals. His isolation of sodium and potassium represented a significant departure from the conventional method of classifying metals solely based on their ponderousness or high densities. Sodium and potassium, on the contrary, floated on water. Nevertheless, their classification as metals was firmly established by their distinct chemical properties.
As early as 1811, attempts were initiated to enhance the differentiation between metals and nonmetals by examining a range of properties, including physical, chemical, and electron-related characteristics. The table provided here outlines 22 such properties, sorted by type and year of mention.
One of the most commonly recognized properties used in this context is the effect of heating on electrical conductivity. As temperature rises, the conductivity of metals decreases while that of nonmetals increases. However, plutonium, carbon, arsenic, and antimony defy the norm. When plutonium (a metal) is heated within a temperature range of −175 to +125 °C its conductivity increases. Similarly, despite its common classification as a nonmetal, when carbon (as graphite) is heated it experiences a decrease in electrical conductivity. Arsenic and antimony, which are occasionally classified as nonmetals, show behavior similar to carbon, highlighting the complexity of the distinction between metals and nonmetals.
Kneen and colleagues proposed that the classification of nonmetals can be achieved by establishing a single criterion for metallicity. They acknowledged that various plausible classifications exist and emphasized that while these classifications may differ to some extent, they would generally agree on the categorization of nonmetals.
However, Emsley pointed out the complexity of this task, asserting that no single property alone can unequivocally assign elements to either the metal or nonmetal category. Furthermore, Jones emphasized that classification systems typically rely on more than two attributes to define distinct types.
An approach to distinguishing between metallic and nonmetallic properties was suggested by Johnson, emphasizing the significance of physical properties, while acknowledging the potential need for other properties in certain ambiguous cases. His observations highlighted several key distinctions:
- Physical state—Elements that exist as gases or are nonconductors are typically classified as nonmetals.
- Solid nonmetals—Solid nonmetals exhibit characteristics such as hardness and brittleness (B, Si, Ge) or softness and crumbliness, setting them apart from metals that are generally malleable and ductile.
- Chemical behavior—Nonmetal oxides tend to be acidic, providing another useful criterion for identifying nonmetals.
Several authors have noted that, in general, and among other properties, nonmetals have low densities and high electronegativity, which is consistent with the data presented in the table. Nonmetallic elements are predominantly located in the top right quadrant of this table, where density is low and electronegativity values are relatively high. In contrast, the other three quadrants are primarily occupied by metals. Goldwhite and Spielman added that, "... lighter elements tend to be more electronegative than heavier ones." The average electronegativity for the elements in the table with densities less than 7 gm/cm3 (metals and nonmetals) is 2.09 compared to 1.68 for the metals having densities of more than 7 gm/cm3.
While some authors choose to further subdivide elements into metals, metalloids, and nonmetals, Oderberg disagrees with this approach. He argues that according to the principles of categorization, anything not classified as a metal should be considered a nonmetal.
Development of types

In 1844, Alphonse Dupasquier , a French doctor, pharmacist, and chemist, established a basic taxonomy of nonmetals to aid in the study of these elements. He wrote:
- They will be divided into four groups or sections, as in the following:
- Organogens O, N, H, C
- Sulphuroids S, Se, P
- Chloroides F, Cl, Br, I
- Boroids B, Si.
Dupasquier's fourfold classification has echoes in the modern types of nonmetals. The organogens and sulphuroids are akin to the unclassified nonmetals. The chloroide nonmetals were later recognized independently as halogens. The boroid nonmetals eventually evolved into the metalloids, with this classification beginning as early as 1864. The noble gases were also identified as a distinct group among the nonmetals, dating back to as early as 1900.
Comparison of selected properties
The two tables in this section list some physical and chemical properties of metals and those of the three to four types of nonmetals, based on the most stable forms of the elements in ambient conditions.
The aim is to show that most properties display a left-to-right progression in metallic-to-nonmetallic character or average values. Some overlapping of boundaries can occur as outlier elements of each type exhibit less-distinct, hybrid-like, or atypical properties. These overlaps or transitional points, along with horizontal, diagonal, and vertical relationships between the elements, form part of the "great deal of information" summarized by the periodic table.
The dashed lines around the columns for metalloids signify that the treatment of these elements as a distinct type can vary depending on the author, or classification scheme in use.
Physical
Physical properties are presented in loose order of ease of their determination.
|
Element type | ||||
---|---|---|---|---|---|
Property | Metals | Metalloids | Unc. nonmetals | Halogen nonmetals | Noble gases |
Form and density | solid | solid | solid or gas | solid, liquid or gas | gas |
often high density such as Fe, Pb, W | low to moderately high density | low density | low density | low density | |
some light metals including Be, Mg, Al | all lighter than Fe | H, N lighter than air | He, Ne lighter than air | ||
Appearance | lustrous | lustrous |
|
|
colorless |
Elasticity | mostly malleable and ductile (Hg is liquid) | brittle | C, black P, S, Se brittle | iodine is brittle | not applicable |
Electrical conductivity | good |
|
|
|
poor |
Electronic structure | metallic (Bi is a semimetal) | semimetal (As, Sb) or semiconductor |
|
semiconductor (I) or insulator | insulator |
Chemical
Chemical properties start with general characteristics and proceed to more specific details.
|
Element type | ||||
---|---|---|---|---|---|
Property | Metals | Metalloids | Unc. nonmetals | Halogen nonmetals | Noble gases |
General chemical behavior |
|
weakly nonmetallic | moderately nonmetallic | strongly nonmetallic |
|
Oxides | basic; some amphoteric or acidic | amphoteric or weakly acidic | acidic or neutral | acidic | metastable XeO3 is acidic; stable XeO4 strongly so |
few glass formers | all glass formers | some glass formers | no glass formers reported | no glass formers reported | |
ionic, polymeric, layer, chain, and molecular structures | polymeric in structure |
|
|
| |
Compounds with metals | alloys or intermetallic compounds | tend to form alloys or intermetallic compounds |
|
mainly ionic | simple compounds in ambient conditions not known |
Ionization energy (kJ mol−1) ‡ | low to high | moderate | moderate to high | high | high to very high |
376 to 1,007 | 762 to 947 | 941 to 1,402 | 1,008 to 1,681 | 1,037 to 2,372 | |
average 643 | average 833 | average 1,152 | average 1,270 | average 1,589 | |
Electronegativity (Pauling) ‡ | low to high | moderate | moderate to high | high | high (Rn) to very high |
0.7 to 2.54 | 1.9 to 2.18 | 2.19 to 3.44 | 2.66 to 3.98 | ca. 2.43 to 4.7 | |
average 1.5 | average 2.05 | average 2.65 | average 3.19 | average 3.3 |
† Hydrogen can also form alloy-like hydrides
‡ The labels low, moderate, high, and very high are arbitrarily based on the value spans listed in the table