Long considered a joke, or a pipe dream, fusion is suddenly making enormous leaps.
The
idea first lit up Dennis Whyte when he was in high school, in the
remote reaches of Saskatchewan, Canada, in the 1980s. He wrote a term
paper on how scientists were trying to harness fusion (the physical
effect that fuels the stars) in wondrously efficient power plants on
Earth. This is the ultimate clean-energy dream. It would provide massive
amounts of clean electricity, with no greenhouse gases or air
pollution. It would do it on a constant basis, unlike solar and wind.
Whatever waste it created would be easily manageable, unlike today’s
nuclear power plants. And fuel would be limitless. One of the main
ingredients needed for fusion is abundant in water. Just one little gram
of hydrogen fuel for a fusion reactor would provide as much power as 10
tons of coal.
Whyte
got an A on that paper, but his physics teacher also wrote: “It’s too
complicated.” That comment, Whyte says with a hearty laugh, “was sort of
a harbinger of things to come.”
Indeed,
over the next few decades, as Whyte mastered the finicky physics that
fusion power would require and became a professor at MIT, the concept
seemingly got no closer to becoming reality. It’s not that the science
was shaky: It’s that reliably bottling up miniature stars, inside
complex machines on Earth, demands otherworldly amounts of patience, not
to mention billions and billions of dollars. Researchers, like Whyte, knew all too well the sardonic joke about their work: fusion is the energy source of the future, and it always will be.
That
line took on an especially bitter edge one day in 2012, when the U.S.
Department of Energy announced it would eliminate funding for MIT’s
experimental fusion reactor. Whyte was angry about the suddenness of the
news. “It was absolutely absurd — you can put that in your
article — fucking absurd that
happened with a program that was acknowledged to be excellent.” But
above all, he was dismayed. Global warming was bearing down year after
year, yet this idea that could save civilization was losing what little
momentum it had.
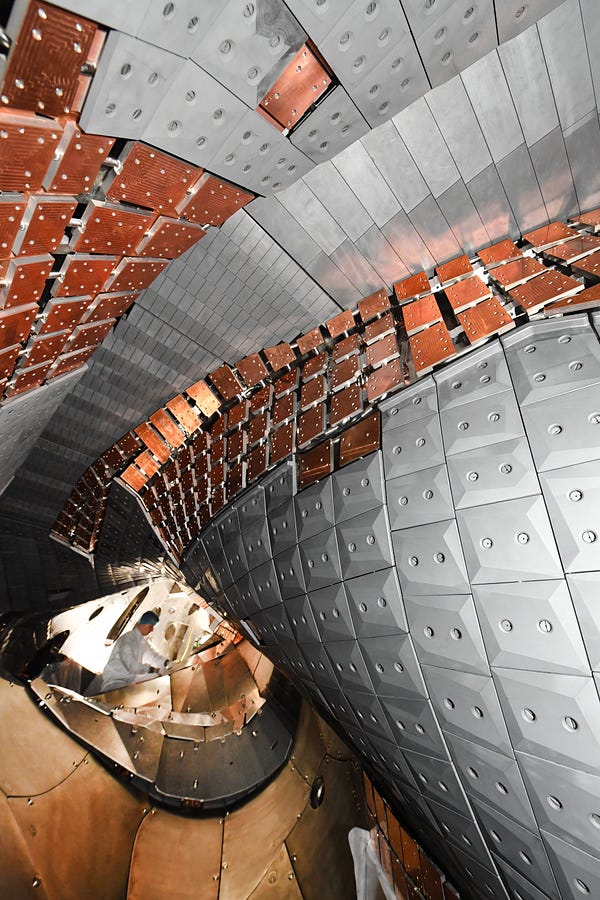
So
Whyte thought about giving up. He looked for other things to focus on,
“stuff that wasn’t as exciting, quite frankly,” but stuff that would be
achievable. “Everyone understands delays in projects, and science
hurdles you’ve got to overcome, but I saw fusion energy being used for
something accelerating away from us,” he says. “You start getting pretty
dejected when you realize, in your professional career, you’re never
going to see this happen.”
As
it turned out, Whyte never really walked away. Instead, he and his
colleagues and graduate students at MIT’s Plasma Science and Fusion
Center figured out a new angle. And last winter, MIT declared that
Whyte’s lab had a fundamentally new approach to fusion and threw its weight behind their plan
with an unusually public bet, spinning out a company to capitalize on
it. An Italian oil company and private investors — including a firm funded by Bill Gates and Jeff Bezos — put
at least $75 million into the company, known as Commonwealth Fusion
Systems [CFS]. The startup intends to demonstrate the workings of fusion
power by 2025.
The recent progress is remarkable, says the founder of one startup developing fusion power. “The world has been waiting for fusion for a long time.”
Real,
live, economically viable power plants could then follow in the 2030s.
No joke. When I ask Whyte, who is 54, to compare his level of optimism
now to any other point in his career, he says, simply: “It is at the
maximum.”
But
it’s not just MIT. At least 10 other startups also are trying new
approaches to fusion power. All of them contend that it’s no longer a
tantalizingly tricky science experiment, and is becoming a matter of
engineering. If even just one of these ventures can pull it off, the
energy source of the future is closer than it seems.
“It’s
remarkable,” says David Kingham, executive vice chairman of Tokamak
Energy, a British company whose goal is to put fusion power on the grid
by 2030. “The world has been waiting for fusion for a long time.”
Imagine
that I told you I was developing a special machine. If I put power into
it, I could get 10 times as much out. Because of the undeniable laws of
physics, I could show you on paper exactly why it should be a
cost-effective source of vast amounts of electricity.
Oh,
here’s the catch: My paper sketch would come true — especially the part
about it being cost-effective — but only if I built the machine just
right. Which might require materials that haven’t been invented yet.
Until I perfected that design, my machine would use up more power than
it produced.
And I couldn’t get close to perfecting the design without
spending years and years building expensive test machines that would
reveal problems that I would try to address in subsequent versions.
If it seems crazy, well, that’s the story of fusion power.
Fusion
definitely works. You see it every day. Our sun and other stars blast
hydrogen atoms together with such intense force that their nuclei
overcome their normal inclination to repel each other. Instead they
fuse, sparking a reaction that transforms the hydrogen into helium and
releases cosmic amounts of energy in the process.
We
also have great paper sketches for fusion power machines. Fusion
happens inside stars because of the crushing pressure created by their
gravity. To generate that effect inside a fusion reactor, ionized
gas — which is called plasma — must be heated and compressed by man-made
forces, such as an ultra-powerful magnetic field. But whatever the
method, there’s just one main goal. If you get enough plasma to stay hot
enough for long enough, then you can trigger so much fusion inside it
that a huge multiplier effect is unlocked. At that point, the energy
that is released helps keep the plasma hot, extending the reaction. And
there still is plenty of energy left over to turn into electricity.
The
problem is that we’re still plugging away on predecessors to the
machines that could generate that effect. Ever since the 1950s,
scientists have used spherical or doughnut-shaped machines called
tokamaks, including the one at MIT that lost funding a few years ago, to
create fusion reactions in plasmas bottled up by magnetic fields. But
no one has done it long enough — while also getting it hot enough and
dense enough — to really tip the balance and get it going. Heating the
plasma and squeezing it in place still takes more energy than you can
harvest from it.
So,
that’s the name of the game in fusion: to get past that point. ITER, a
mega-billion-dollar reactor being built in France by an international
consortium, is designed to do it and finally prove the concept. But
ITER — which is also way behind schedule and over budget — overcomes the
limitations of previous tokamaks by being enormous. It’s the size of 60
soccer fields, which probably isn’t an economical setup for power
plants that the world will need by the tens of thousands.
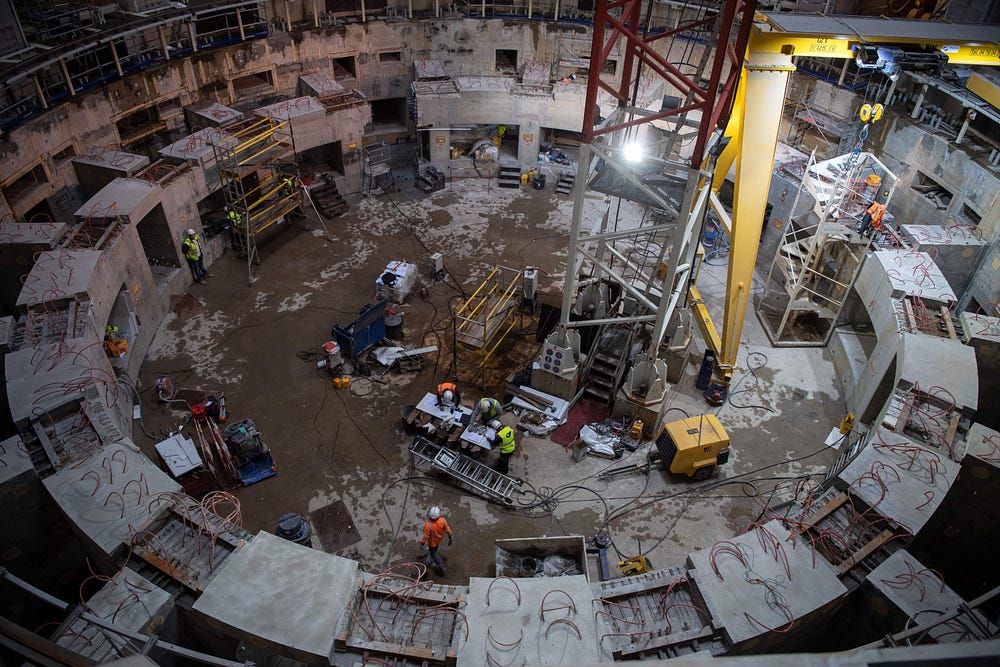
Could
you go the other direct
ion, and instead make fusion machines much
smaller, which is also to say much less expensive? That is what
motivates all the fusion startups. Several have decided the answer is to
use something other than a tokamak and its circular coils of magnets.
They’re updating old designs, including hitting plasma with lasers, or
cooking up new ones, such as compressing it with something like a particle accelerator. One startup plans to push on the material with pistons.
But
Whyte and his colleagues at MIT made a different decision, one that
could prove crucial to making fusion power arise sooner than people
expect. Even though things looked dire a few years ago, when their
fusion machine lost funding, Whyte’s team decided to double down on
tokamaks. As Whyte saw it, why try to invent something totally new when
you could take advantage of all those decades and billions spent
researching tokamaks? Instead, they would rethink the design to make
tokamaks modular and much cheaper and weave in brand-new materials that
can induce and confine a fusion reaction.
After
getting the news of the funding shutdown, the university, and other
supporters of the program, persuaded Congress to grant a temporary
reprieve. They could keep running their fusion reactor into 2016, enough
time for experiments to be finished and to keep PhD students going on
the research they had come to MIT to undertake. And then they dug in.
The
most intriguing questions Whyte and his students were exploring had to
do with how tokamaks could produce lots of electricity without being
gigantic and expensive. MIT’s tokamak, which still sits in a two-story
tall, garage-like room in a former Nabisco cookie warehouse, generated a
magnetic field by running electricity through copper coils that
surrounded a round metal chamber. In that chamber, plasma would be
heated with microwaves and other methods to millions of degrees. On one of its last runs, it set a new record for plasma pressure while hitting 35 million Celsius.
Just
outside the chamber, the vital measurement isn’t heat, but cold. The
magnets that squeeze the plasma in place have to be kept well below
minus-200 Celsius, or else their performance will degrade from a buildup
of electrical resistance.
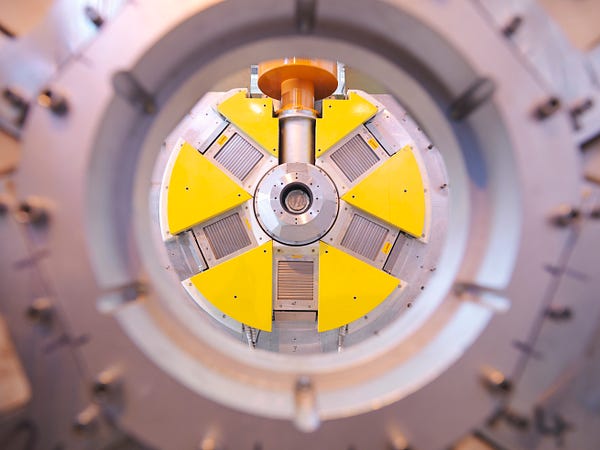
It
was a graduate student who suggested that the MIT team see what would
happen if they made magnets out of a newly developed superconducting
tape. A superconductor conducts electricity so well that it doesn’t
build up electrical resistance and this new tape maintains that
property, even at slightly higher temperatures than other
superconductors do.
Using
less energy on cooling could make a tokamak cheaper to run. But that
benefit was minor compared to the other things Whyte’s group figured
out. As they plotted out ways of winding the tape into coils in a
tokamak, they realized this method could double the strength of the
magnetic field they could exert on a plasma. Increasing the field
strength is crucial because plasma is wild. It’s unstable and evasive,
and only overwhelming force can keep it from spreading out and cooling
too much.
Perhaps best of all: using this tape instead of rigid superconductors could make the machine 10 times smaller.
That
led them to another problem with traditional tokamaks. If you need to
replace parts of the machine, you have to take the whole thing apart and
put it back together. That’s unacceptable for a power plant in regular
use. And again, one of Whyte’s graduate students had a great idea. If
you apply the superconducting tape in sections, with joints, the magnets
can be snapped on and off for quick and easy repairs or upgrades.
“This was the beginning of the ‘aha!’ moment,” Whyte says. “The people who are in CFS were in that class.”
Other
big ideas kept coming. One of the great things about fusion is its
inherent safety. It’s impossible for this tiny star to slip out and
cause trouble, because the plasma’s weird physical state can’t be
sustained outside of the magnetic field. Still, the plasma does send
something out that you’ve got to deal with: neutrons.
Fusion
projects generally aim to fuse two forms of hydrogen: deuterium and
tritium. Deuterium is readily available in seawater, but tritium is very
rare, so you have to make it. (More on that in a minute.) In this
version of fusion, 80 percent of the energy that is released comes out
in the form of neutrons. These are subatomic particles that have no
electric charge, so they’re not contained by the magnetic field. They
come flying out like angry spittle.
In
fusion experiments measured in seconds or less, flying neutrons aren’t a
big problem. But over time, they can be nasty. These particles jump a
foot and a half from the plasma and have enough energy to rearrange the
atoms in the tokamak’s inner wall, eventually degrading it. What to do
about that in a power plant that needs to run for long stretches?
Whyte describes the answer with a wry smile. “We turned the problem around,” he says.
In
essence, the MIT plan takes a ride on the neutrons by catching them in a
liquid. Neutrons wreck solid materials by scrambling the order of their
atoms, but liquids are already disordered, by definition. In the design
that CFS is developing, the neutrons pass through an inch or two of
steel and then barrel into a liquified salt, which they essentially just
heat up. Then, that molten salt can be pumped around a power station to
generate electricity. By the way, there’s lithium in the molten salt,
and when neutrons hit lithium, they create tritium, which you can take
out and use to fuel the fusion reactor.
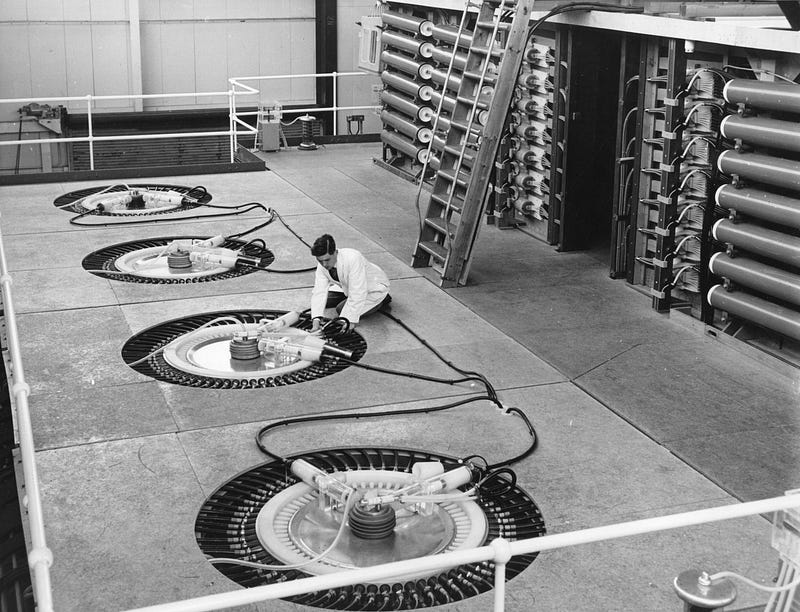
This
setup isn’t perfect, however. Blanketing the tokamak’s steel wall with
molten salt will lessen, but not eliminate, the damage that the neutrons
would otherwise cause to the metal. It will have to be replaced every
so often. Just how often? That’s a crucial question for the cost of a
power plant.
For
now, Whyte says, the metal barrier should last a year or two. That’s
not great, so materials that better withstand neutrons have to be
developed, to extend the lifespan of that wall. That looks doable;
reducing the erosion of the wall in fusion reactors is a long-standing
field of research.
But
the issue is nonetheless significant enough that General Fusion, the
company that intends to compress plasma with pistons, plans to keep a
solid metal case relatively far away. It will directly surround the
plasma with liquid metal that gets pumped off to convert its heat to
electricity. There will be lithium in that liquid, too, to breed
tritium.
Even
if the MIT team manages to extend the life of the barrier, there’s
another issue: The neutron bombardment will eventually render the metal
radioactive.
Is
that a big problem? Well, one of the novel things about a fusion
company called TAE Technologies, which has raised $600 million from
Google, the late Microsoft founder Paul Allen, and other luminaries, is
that it plans to fuse hydrogen protons with boron, a reasonably abundant
element, because that reaction emits hardly any neutrons. TAE’s
co-founder and CEO, Michl Binderbauer, says that because of its cleaner
profile, hydrogen-boron fusion is “the single shining opportunity for
mankind.”
But
since we’re talking about fusion, of course there’s a catch.
Hydrogen-boron fusion is much harder to pull off: The plasma has to get
to billions of degrees, not millions. And the “reaction rate” is much
lower, which means less fusion happens. TAE is going to start with
deuterium-tritium fusion before trying to work its way up.
In
the meantime, Whyte and just about everyone else in fusion thinks
deuterium-tritium fusion is well worth gunning for. Any radioactive
components in MIT’s design will be relatively small and have a short
half-life. The material would be nowhere near as problematic as the
stuff that comes out of nuclear power plants today. If fusion plant
operators have to replace the inner wall from the reactor Whyte
envisions, they’d “put it in a swimming pool for 10 years,” he says.
“And then you can walk up beside it.”
Before any of that happens, CFS will try to pull off fusion’s most elusive trick: doing something ahead of schedule.
With
the investment it’s raised, the company has about three years to test
components of its reactor design, especially those still-unproven new
magnets. Then, it will need to raise hundreds of millions to build a
prototype reactor, at a location to be determined. The company has said
it intends to get that reactor running by 2025. But its CEO, a former
MIT graduate student named Robert Mumgaard, says it could happen even
sooner.
Alas,
fusion timelines still have a habit of slipping, even in private
companies. Over the past few years, the defense contractor Lockheed
Martin, and a few startups, said they hoped to show working prototypes
by now — possibly even ones that achieved the ultimate, a net power
gain. That hasn’t happened. When I asked for updates, I got some vague
replies, ranging from “we are hard at work” to “the preliminary results
are promising.”
If fusion power just won’t work, “I’m scared for the world,” says the CEO of Commonwealth Fusion Systems.
I got the most reassuring answer from Christofer Mowry, CEO of General Fusion.
His
company said in 2017 that it hoped to get its first prototype running
in three to five years. It’s really more like five years from now. But,
he says, that’s because the company has needed time to raise “a few
hundred million dollars,” not because fusion science is still iffy.
Because so many companies are trying to make fusion power practical, and
because demand for it will be so high, “I’m 100 percent confident that
this is going to happen,” Mowry says. “Are we going to have commercial
fusion power plants on the grid by 2030? Maybe. But it won’t be 50
years, I can tell you that.”
At
CFS, Mumgaard sees parallels with the story of human flight. Before the
Wright brothers finally got a plane off the ground, a lot of people
tried and got kind of close. Plenty of observers assumed that meant
human flight would always remain a fantasy. But all that time, through
all those failures with gliders and flapping man-made wings, engineers
were systematically probing aerodynamics. The Wright brothers built on
that knowledge and combined it with insights about control mechanisms
that they had from working with bicycles. And only then, was it obvious:
yes, humans can fly.
“When you have the insight into a piece of technology and you get it over that hump, it goes,”
Mumgaard says. “We’re think we’re at this point.” He refers to his
company’s plan to build a prototype as the Kitty Hawk moment.
But
what if that parallel breaks down? What if fusion power just won’t
work, or won’t work at a cost that anyone will be willing to pay? Then
“I’m scared for the world,” Mumgaard says.
And
it’s hard to cheer him up on that point. None of the existing
carbon-free alternatives seem suited to the scale of the climate
problem. Conventional nuclear power is unpopular and expensive. There
aren’t many more waterways to dam. For solar and wind to be the primary
answer, you’d need epic amounts of batteries, which might be
environmentally or economically prohibitive.
When
I pose the same question to Whyte, I get a slightly different answer.
He sounds like a person who has considered what would happen if he gave
up on this dream, and then renewed it instead. “I would never say ‘if we
don’t develop fusion we’re not going to make it,’” he says. “But, boy,
I’ll put it the other way around: If you make fusion economical, you
have given yourself an arrow in the quiver which is almost unmatched in
going after this.”
“We’re
giving it our best shot,” he continues. “Others are giving it their
best shot.” And then he slaps his hand on the table in front of him for
emphasis. “Let’s get there.”